Integrated Design of a Membrane-Lytic Peptide-Based Intravenous Nanotherapeutic Suppresses Triple-Negative Breast Cancer
- PMID: 35246961
- PMCID: PMC9069370
- DOI: 10.1002/advs.202105506
Integrated Design of a Membrane-Lytic Peptide-Based Intravenous Nanotherapeutic Suppresses Triple-Negative Breast Cancer
Abstract
Membrane-lytic peptides offer broad synthetic flexibilities and design potential to the arsenal of anticancer therapeutics, which can be limited by cytotoxicity to noncancerous cells and induction of drug resistance via stress-induced mutagenesis. Despite continued research efforts on membrane-perforating peptides for antimicrobial applications, success in anticancer peptide therapeutics remains elusive given the muted distinction between cancerous and normal cell membranes and the challenge of peptide degradation and neutralization upon intravenous delivery. Using triple-negative breast cancer as a model, the authors report the development of a new class of anticancer peptides. Through function-conserving mutations, the authors achieved cancer cell selective membrane perforation, with leads exhibiting a 200-fold selectivity over non-cancerogenic cells and superior cytotoxicity over doxorubicin against breast cancer tumorspheres. Upon continuous exposure to the anticancer peptides at growth-arresting concentrations, cancer cells do not exhibit resistance phenotype, frequently observed under chemotherapeutic treatment. The authors further demonstrate efficient encapsulation of the anticancer peptides in 20 nm polymeric nanocarriers, which possess high tolerability and lead to effective tumor growth inhibition in a mouse model of MDA-MB-231 triple-negative breast cancer. This work demonstrates a multidisciplinary approach for enabling translationally relevant membrane-lytic peptides in oncology, opening up a vast chemical repertoire to the arms race against cancer.
Keywords: anticancer peptides; drug resistance; membrane-active anticancer agents; multicellular tumor spheroids; murine models; nanoparticles; triple negative breast cancer.
© 2022 The Authors. Advanced Science published by Wiley-VCH GmbH.
Conflict of interest statement
The authors declare no conflict of interest.
Figures
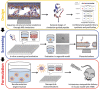
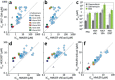
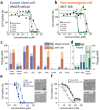
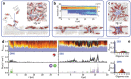
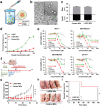
Similar articles
-
Lipid nanocarriers of a lipid-conjugated estrogenic derivative inhibit tumor growth and enhance cisplatin activity against triple-negative breast cancer: pharmacokinetic and efficacy evaluation.Mol Pharm. 2015 Apr 6;12(4):1105-20. doi: 10.1021/mp5008629. Epub 2015 Mar 2. Mol Pharm. 2015. PMID: 25661724
-
[10]-Gingerol improves doxorubicin anticancer activity and decreases its side effects in triple negative breast cancer models.Cell Oncol (Dordr). 2020 Oct;43(5):915-929. doi: 10.1007/s13402-020-00539-z. Epub 2020 Aug 6. Cell Oncol (Dordr). 2020. PMID: 32761561
-
Albumin Nanoparticles Surface Decorated with a Tumor-Homing Peptide Help in Selective Killing of Triple-Negative Breast Cancer Cells.ACS Appl Mater Interfaces. 2023 Oct 11;15(40):46721-46737. doi: 10.1021/acsami.3c11561. Epub 2023 Sep 27. ACS Appl Mater Interfaces. 2023. PMID: 37756635
-
Highly Efficient Photoswitchable Smart Polymeric Nanovehicle for Gene and Anticancer Drug Delivery in Triple-Negative Breast Cancer.ACS Biomater Sci Eng. 2024 Apr 8;10(4):2299-2323. doi: 10.1021/acsbiomaterials.4c00115. Epub 2024 Mar 29. ACS Biomater Sci Eng. 2024. PMID: 38551335
-
Doxorubicin-Based Hybrid Compounds as Potential Anticancer Agents: A Review.Molecules. 2022 Jul 13;27(14):4478. doi: 10.3390/molecules27144478. Molecules. 2022. PMID: 35889350 Free PMC article. Review.
Cited by
-
Masking the transmembrane region of the amyloid β precursor protein as a safe means to lower amyloid β production.Alzheimers Dement (N Y). 2023 Nov 8;9(4):e12428. doi: 10.1002/trc2.12428. eCollection 2023 Oct-Dec. Alzheimers Dement (N Y). 2023. PMID: 37954165 Free PMC article.
-
The role of peptides in reversing chemoresistance of breast cancer: current facts and future prospects.Front Pharmacol. 2023 May 22;14:1188477. doi: 10.3389/fphar.2023.1188477. eCollection 2023. Front Pharmacol. 2023. PMID: 37284316 Free PMC article. Review.
-
Perturbing plasma membrane lipid: a new paradigm for tumor nanotherapeutics.Theranostics. 2023 Apr 23;13(8):2471-2491. doi: 10.7150/thno.82189. eCollection 2023. Theranostics. 2023. PMID: 37215569 Free PMC article. Review.
-
Nanobiotherapeutic strategies to target immune microenvironment of triple-negative breast cancer.Am J Cancer Res. 2022 Sep 15;12(9):4083-4102. eCollection 2022. Am J Cancer Res. 2022. PMID: 36225648 Free PMC article. Review.
-
Synthesis and Discovery of Ligustrazine-Heterocycle Derivatives as Antitumor Agents.Front Chem. 2022 Jul 25;10:941367. doi: 10.3389/fchem.2022.941367. eCollection 2022. Front Chem. 2022. PMID: 35958230 Free PMC article.
References
-
- a) Marx J., Science 2007, 317, 1029; - PubMed
- b) Nguyen L. V., Vanner R., Dirks P., Eaves C. J., Nat. Rev. Cancer 2012, 12, 133; - PubMed
- c) Pattabiraman D. R., Weinberg R. A., Nat. Rev. Drug Discovery 2014, 13, 497; - PMC - PubMed
- d) Plaks V., Kong N., Werb Z., Cell Stem Cell 2015, 16, 225; - PMC - PubMed
- e) Kaiser J., Science 2015, 347, 226. - PubMed
-
- Dean M., Fojo T., Bates S., Nat. Rev. Cancer 2005, 5, 275. - PubMed
-
- Chen C. H., Lu T. K., Antibiotics 2020, 9, 24.
Publication types
MeSH terms
Substances
Grants and funding
LinkOut - more resources
Full Text Sources
Miscellaneous