Sterol Metabolism Differentially Contributes to Maintenance and Exit of Quiescence
- PMID: 35237594
- PMCID: PMC8882848
- DOI: 10.3389/fcell.2022.788472
Sterol Metabolism Differentially Contributes to Maintenance and Exit of Quiescence
Abstract
Nutrient starvation initiates cell cycle exit and entry into quiescence, a reversible, non-proliferative state characterized by stress tolerance, longevity and large-scale remodeling of subcellular structures. Depending on the nature of the depleted nutrient, yeast cells are assumed to enter heterogeneous quiescent states with unique but mostly unexplored characteristics. Here, we show that storage and consumption of neutral lipids in lipid droplets (LDs) differentially impacts the regulation of quiescence driven by glucose or phosphate starvation. Upon prolonged glucose exhaustion, LDs were degraded in the vacuole via Atg1-dependent lipophagy. In contrast, yeast cells entering quiescence due to phosphate exhaustion massively over-accumulated LDs that clustered at the vacuolar surface but were not engulfed via lipophagy. Excessive LD biogenesis required contact formation between the endoplasmic reticulum and the vacuole at nucleus-vacuole junctions and was accompanied by a shift of the cellular lipid profile from membrane towards storage lipids, driven by a transcriptional upregulation of enzymes generating neutral lipids, in particular sterol esters. Importantly, sterol ester biogenesis was critical for long-term survival of phosphate-exhausted cells and supported rapid quiescence exit upon nutrient replenishment, but was dispensable for survival and regrowth of glucose-exhausted cells. Instead, these cells relied on de novo synthesis of sterols and fatty acids for quiescence exit and regrowth. Phosphate-exhausted cells efficiently mobilized storage lipids to support several rounds of cell division even in presence of inhibitors of fatty acid and sterol biosynthesis. In sum, our results show that neutral lipid biosynthesis and mobilization to support quiescence maintenance and exit is tailored to the respective nutrient scarcity.
Keywords: NVJ; lipid droplets; lipophagy; membrane contact sites; quiescence; sterol ester; sterols; yeast.
Copyright © 2022 Peselj, Ebrahimi, Broeskamp, Prokisch, Habernig, Alvarez-Guerra, Kohler, Vögtle and Büttner.
Conflict of interest statement
The authors declare that the research was conducted in the absence of any commercial or financial relationships that could be construed as a potential conflict of interest.
Figures
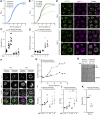
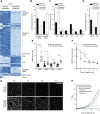
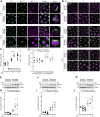
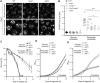
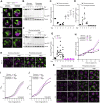
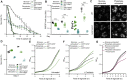
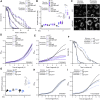
Similar articles
-
Stationary phase lipophagy as a cellular mechanism to recycle sterols during quiescence.Autophagy. 2014;10(11):2075-6. doi: 10.4161/auto.36137. Autophagy. 2014. PMID: 25484090 Free PMC article.
-
LDO proteins and Vac8 form a vacuole-lipid droplet contact site to enable starvation-induced lipophagy in yeast.Dev Cell. 2024 Mar 25;59(6):759-775.e5. doi: 10.1016/j.devcel.2024.01.014. Epub 2024 Feb 13. Dev Cell. 2024. PMID: 38354739
-
Endoplasmic Reticulum-Vacuole Contact Sites "Bloom" With Stress-Induced Lipid Droplets.Contact (Thousand Oaks). 2018 Jan-Dec;1:10.1177/2515256418756112. doi: 10.1177/2515256418756112. Epub 2018 Apr 3. Contact (Thousand Oaks). 2018. PMID: 30112463 Free PMC article.
-
The ménage à trois of autophagy, lipid droplets and liver disease.Autophagy. 2022 Jan;18(1):50-72. doi: 10.1080/15548627.2021.1895658. Epub 2021 Apr 2. Autophagy. 2022. PMID: 33794741 Free PMC article. Review.
-
Lipid Droplets and Their Autophagic Turnover via the Raft-Like Vacuolar Microdomains.Int J Mol Sci. 2021 Jul 29;22(15):8144. doi: 10.3390/ijms22158144. Int J Mol Sci. 2021. PMID: 34360917 Free PMC article. Review.
Cited by
-
Enhanced secretion of promyogenic exosomes by quiescent muscle cells.Front Cell Dev Biol. 2024 Jul 23;12:1381357. doi: 10.3389/fcell.2024.1381357. eCollection 2024. Front Cell Dev Biol. 2024. PMID: 39108837 Free PMC article.
-
A Systematic Review on Quiescent State Research Approaches in S. cerevisiae.Cells. 2023 Jun 12;12(12):1608. doi: 10.3390/cells12121608. Cells. 2023. PMID: 37371078 Free PMC article. Review.
References
Grants and funding
LinkOut - more resources
Full Text Sources
Molecular Biology Databases
Research Materials