Grxcr1 regulates hair bundle morphogenesis and is required for normal mechanoelectrical transduction in mouse cochlear hair cells
- PMID: 35235570
- PMCID: PMC8890737
- DOI: 10.1371/journal.pone.0261530
Grxcr1 regulates hair bundle morphogenesis and is required for normal mechanoelectrical transduction in mouse cochlear hair cells
Abstract
Tasmanian devil (tde) mice are deaf and exhibit circling behaviour. Sensory hair cells of mutants show disorganised hair bundles with abnormally thin stereocilia. The origin of this mutation is the insertion of a transgene which disrupts expression of the Grxcr1 (glutaredoxin cysteine rich 1) gene. We report here that Grxcr1 exons and transcript sequences are not affected by the transgene insertion in tde homozygous (tde/tde) mice. Furthermore, 5'RACE PCR experiments showed the presence of two different transcripts of the Grxcr1 gene, expressed in both tde/tde and in wild-type controls. However, quantitative analysis of Grxcr1 transcripts revealed a significantly decreased mRNA level in tde/tde mice. The key stereociliary proteins ESPN, MYO7A, EPS8 and PTPRQ were distributed in hair bundles of homozygous tde mutants in a similar pattern compared with control mice. We found that the abnormal morphology of the stereociliary bundle was associated with a reduction in the size and Ca2+-sensitivity of the mechanoelectrical transducer (MET) current. We propose that GRXCR1 is key for the normal growth of the stereociliary bundle prior to the onset of hearing, and in its absence hair cells are unable to mature into fully functional sensory receptors.
Conflict of interest statement
The authors have declared that no competing interests exist.
Figures
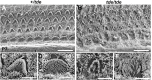
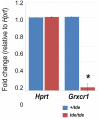
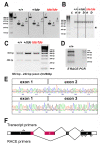
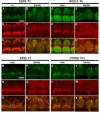
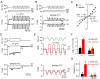
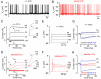
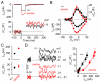
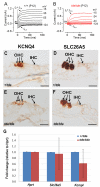
Similar articles
-
Grxcr1 Promotes Hair Bundle Development by Destabilizing the Physical Interaction between Harmonin and Sans Usher Syndrome Proteins.Cell Rep. 2018 Oct 30;25(5):1281-1291.e4. doi: 10.1016/j.celrep.2018.10.005. Cell Rep. 2018. PMID: 30380418 Free PMC article.
-
Loss of Baiap2l2 destabilizes the transducing stereocilia of cochlear hair cells and leads to deafness.J Physiol. 2021 Feb;599(4):1173-1198. doi: 10.1113/JP280670. Epub 2020 Nov 26. J Physiol. 2021. PMID: 33151556 Free PMC article.
-
Grxcr2 is required for stereocilia morphogenesis in the cochlea.PLoS One. 2018 Aug 29;13(8):e0201713. doi: 10.1371/journal.pone.0201713. eCollection 2018. PLoS One. 2018. PMID: 30157177 Free PMC article.
-
Stereocilia morphogenesis and maintenance through regulation of actin stability.Semin Cell Dev Biol. 2017 May;65:88-95. doi: 10.1016/j.semcdb.2016.08.017. Epub 2016 Aug 23. Semin Cell Dev Biol. 2017. PMID: 27565685 Free PMC article. Review.
-
The physiology of mechanoelectrical transduction channels in hearing.Physiol Rev. 2014 Jul;94(3):951-86. doi: 10.1152/physrev.00038.2013. Physiol Rev. 2014. PMID: 24987009 Free PMC article. Review.
Cited by
-
Genome-wide detection of positive and balancing signatures of selection shared by four domesticated rainbow trout populations (Oncorhynchus mykiss).Genet Sel Evol. 2024 Feb 22;56(1):13. doi: 10.1186/s12711-024-00884-9. Genet Sel Evol. 2024. PMID: 38389056 Free PMC article.
References
MeSH terms
Substances
Grants and funding
LinkOut - more resources
Full Text Sources
Molecular Biology Databases
Miscellaneous