Rapid chemically selective 3D imaging in the mid-infrared
- PMID: 35233439
- PMCID: PMC8884451
- DOI: 10.1364/OPTICA.426199
Rapid chemically selective 3D imaging in the mid-infrared
Abstract
The emerging technique of mid-infrared optical coherence tomography (MIR-OCT) takes advantage of the reduced scattering of MIR light in various materials and devices, enabling tomographic imaging at deeper penetration depths. Because of challenges in MIR detection technology, the image acquisition time is, however, significantly longer than for tomographic imaging methods in the visible/near-infrared. Here we demonstrate an alternative approach to MIR tomography with high-speed imaging capabilities. Through femtosecond nondegenerate two-photon absorption of MIR light in a conventional Si-based CCD camera, we achieve wide-field, high-definition tomographic imaging with chemical selectivity of structured materials and biological samples in mere seconds.
Conflict of interest statement
Disclosures. The authors declare no conflicts of interest.
Figures
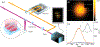
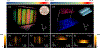
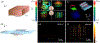
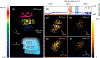
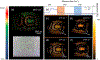
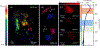
Similar articles
-
Depressing time: Waiting, melancholia, and the psychoanalytic practice of care.In: Kirtsoglou E, Simpson B, editors. The Time of Anthropology: Studies of Contemporary Chronopolitics. Abingdon: Routledge; 2020. Chapter 5. In: Kirtsoglou E, Simpson B, editors. The Time of Anthropology: Studies of Contemporary Chronopolitics. Abingdon: Routledge; 2020. Chapter 5. PMID: 36137063 Free Books & Documents. Review.
-
Single-Exposure, Dual-Energy Subtraction Flat Panel X-Ray Detectors: A Health Technology Assessment.Ont Health Technol Assess Ser. 2024 Nov 12;24(9):1-76. eCollection 2024. Ont Health Technol Assess Ser. 2024. PMID: 39697784 Free PMC article.
-
Far Posterior Approach for Rib Fracture Fixation: Surgical Technique and Tips.JBJS Essent Surg Tech. 2024 Dec 6;14(4):e23.00094. doi: 10.2106/JBJS.ST.23.00094. eCollection 2024 Oct-Dec. JBJS Essent Surg Tech. 2024. PMID: 39650795 Free PMC article.
-
Imaging of ventilation and lung injury with low-frequency tomographic ultrasound.Med Phys. 2024 Dec;51(12):9138-9154. doi: 10.1002/mp.17421. Epub 2024 Sep 23. Med Phys. 2024. PMID: 39312493 Free PMC article.
-
Topical capsaicin (high concentration) for chronic neuropathic pain in adults.Cochrane Database Syst Rev. 2013 Feb 28;(2):CD007393. doi: 10.1002/14651858.CD007393.pub3. Cochrane Database Syst Rev. 2013. Update in: Cochrane Database Syst Rev. 2017 Jan 13;1:CD007393. doi: 10.1002/14651858.CD007393.pub4 PMID: 23450576 Updated. Review.
Cited by
-
Spectral imaging at high definition and high speed in the mid-infrared.Sci Adv. 2022 Nov 18;8(46):eade4247. doi: 10.1126/sciadv.ade4247. Epub 2022 Nov 16. Sci Adv. 2022. PMID: 36383646 Free PMC article.
-
High-speed 2D and 3D mid-IR imaging with an InGaAs camera.APL Photonics. 2021 Sep 1;6(9):096108. doi: 10.1063/5.0061661. Epub 2021 Sep 22. APL Photonics. 2021. PMID: 35498553 Free PMC article.
-
Mid-infrared single-photon 3D imaging.Light Sci Appl. 2023 Jun 9;12(1):144. doi: 10.1038/s41377-023-01179-2. Light Sci Appl. 2023. PMID: 37296123 Free PMC article.
References
-
- Wetzel DL and LeVine SM, “Imaging molecular chemistry with infrared microscopy,” Science 285, 1224 (1999). - PubMed
-
- Wang Y, Wang Y, and Le HQ, “Multi-spectral mid-infrared laser stand-off imaging,” Opt. Express 13, 6572–6586 (2005). - PubMed
-
- Cheung C, Daniel JMO, Tokurakawa M, Clarkson WA, and Liang H, “High resolution Fourier domain optical coherence tomography in the 2 µm wavelength range using a broadband supercontinuum source,” Opt. Express 23, 1992–2001 (2015). - PubMed
-
- Colley CS, Hebden JC, Delpy DT, Cambrey AD, Brown RA, Zibik EA, Ng WH, Wilson LR, and Cockburn JW, “Mid-infrared optical coherence tomography,” Rev. Sci. Instrum 78, 123108 (2007). - PubMed