Forgotten Actors: Glycoside Hydrolases During Elongation Growth of Maize Primary Root
- PMID: 35222452
- PMCID: PMC8866823
- DOI: 10.3389/fpls.2021.802424
Forgotten Actors: Glycoside Hydrolases During Elongation Growth of Maize Primary Root
Abstract
Plant cell enlargement is coupled to dynamic changes in cell wall composition and properties. Such rearrangements are provided, besides the differential synthesis of individual cell wall components, by enzymes that modify polysaccharides in muro. To reveal enzymes that may contribute to these modifications and relate them to stages of elongation growth in grasses, we carried out a transcriptomic study of five zones of the primary maize root. In the initiation of elongation, significant changes occur with xyloglucan: once synthesized in the meristem, it can be linked to other polysaccharides through the action of hetero-specific xyloglucan endotransglycosidases, whose expression boosts at this stage. Later, genes for xyloglucan hydrolases are upregulated. Two different sets of enzymes capable of modifying glucuronoarabinoxylans, mainly bifunctional α-arabinofuranosidases/β-xylosidases and β-xylanases, are expressed in the maize root to treat the xylans of primary and secondary cell walls, respectively. The first set is highly pronounced in the stage of active elongation, while the second is at elongation termination. Genes encoding several glycoside hydrolases that are able to degrade mixed-linkage glucan are downregulated specifically at the active elongation. It indicates the significance of mixed-linkage glucans for the cell elongation process. The possibility that many glycoside hydrolases act as transglycosylases in muro is discussed.
Keywords: RNA-seq; cell wall; elongation (growth); glycoside hydrolase; maize (Zea mays L.); root.
Copyright © 2022 Nazipova, Gorshkov, Eneyskaya, Petrova, Kulminskaya, Gorshkova and Kozlova.
Conflict of interest statement
The authors declare that the research was conducted in the absence of any commercial or financial relationships that could be construed as a potential conflict of interest.
Figures
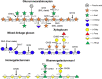
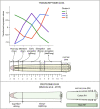
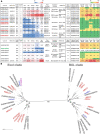
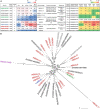
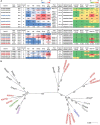
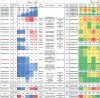
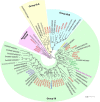
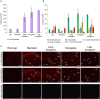
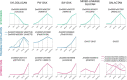
Similar articles
-
Elongating maize root: zone-specific combinations of polysaccharides from type I and type II primary cell walls.Sci Rep. 2020 Jul 2;10(1):10956. doi: 10.1038/s41598-020-67782-0. Sci Rep. 2020. PMID: 32616810 Free PMC article.
-
Arrangement of mixed-linkage glucan and glucuronoarabinoxylan in the cell walls of growing maize roots.Ann Bot. 2014 Oct;114(6):1135-45. doi: 10.1093/aob/mcu125. Epub 2014 Aug 2. Ann Bot. 2014. PMID: 25086589 Free PMC article.
-
Differential expression of α-L-arabinofuranosidases during maize (Zea mays L.) root elongation.Planta. 2015 May;241(5):1159-72. doi: 10.1007/s00425-015-2244-1. Epub 2015 Jan 22. Planta. 2015. PMID: 25608890
-
Enzymatic degradation of xyloglucans by Aspergillus species: a comparative view of this genus.Appl Microbiol Biotechnol. 2021 Apr;105(7):2701-2711. doi: 10.1007/s00253-021-11236-8. Epub 2021 Mar 24. Appl Microbiol Biotechnol. 2021. PMID: 33760931 Review.
-
Plant glycoside hydrolases involved in cell wall polysaccharide degradation.Plant Physiol Biochem. 2006 Jul-Sep;44(7-9):435-49. doi: 10.1016/j.plaphy.2006.08.001. Epub 2006 Sep 7. Plant Physiol Biochem. 2006. PMID: 17023165 Review.
Cited by
-
Elucidating the patterns of pleiotropy and its biological relevance in maize.PLoS Genet. 2023 Mar 21;19(3):e1010664. doi: 10.1371/journal.pgen.1010664. eCollection 2023 Mar. PLoS Genet. 2023. PMID: 36943844 Free PMC article.
-
The evolutionary advantage of an aromatic clamp in plant family 3 glycoside exo-hydrolases.Nat Commun. 2022 Sep 23;13(1):5577. doi: 10.1038/s41467-022-33180-5. Nat Commun. 2022. PMID: 36151080 Free PMC article.
-
Dynamics of cell wall polysaccharides during the elongation growth of rye primary roots.Planta. 2022 Apr 21;255(5):108. doi: 10.1007/s00425-022-03887-2. Planta. 2022. PMID: 35449484
-
Growing Maize Root: Lectins Involved in Consecutive Stages of Cell Development.Plants (Basel). 2022 Jul 7;11(14):1799. doi: 10.3390/plants11141799. Plants (Basel). 2022. PMID: 35890433 Free PMC article.
-
The In Silico Characterization of Monocotyledonous α-l-Arabinofuranosidases on the Example of Maize.Life (Basel). 2023 Jan 18;13(2):266. doi: 10.3390/life13020266. Life (Basel). 2023. PMID: 36836625 Free PMC article.
References
-
- Babcock G. D., Esen A. (1994). Substrate specificity of maize β-glucosidase. Plant Sci. 101 31–39. 10.1016/0168-9452(94)90162-7 - DOI
LinkOut - more resources
Full Text Sources