Correlation Between Fe/S/As Speciation Transformation and Depth Distribution of Acidithiobacillus ferrooxidans and Acidiphilium acidophilum in Simulated Acidic Water Column
- PMID: 35222314
- PMCID: PMC8863614
- DOI: 10.3389/fmicb.2021.819804
Correlation Between Fe/S/As Speciation Transformation and Depth Distribution of Acidithiobacillus ferrooxidans and Acidiphilium acidophilum in Simulated Acidic Water Column
Abstract
It is well known that speciation transformations of As(III) vs. As(V) in acid mine drainage (AMD) are mainly driven by microbially mediated redox reactions of Fe and S. However, these processes are rarely investigated. In this study, columns containing mine water were inoculated with two typical acidophilic Fe/S-oxidizing/reducing bacteria [the chemoautotrophic Acidithiobacillus (At.) ferrooxidans and the heterotrophic Acidiphilium (Aph.) acidophilum], and three typical energy substrates (Fe2+, S0, and glucose) and two concentrations of As(III) (2.0 and 4.5 mM) were added. The correlation between Fe/S/As speciation transformation and bacterial depth distribution at three different depths, i.e., 15, 55, and 105 cm from the top of the columns, was comparatively investigated. The results show that the cell growth at the top and in the middle of the columns was much more significantly inhibited by the additions of As(III) than at the bottom, where the cell growth was promoted even on days 24-44. At. ferrooxidans dominated over Aph. acidophilum in most samples collected from the three depths, but the elevated proportions of Aph. acidophilum were observed in the top and bottom column samples when 4.5 mM As(III) was added. Fe2+ bio-oxidation and Fe3+ reduction coupled to As(III) oxidation occurred for all three column depths. At the column top surfaces, jarosites were formed, and the addition of As(III) could lead to the formation of the amorphous FeAsO4⋅2H2O. Furthermore, the higher As(III) concentration could inhibit Fe2+ bio-oxidation and the formation of FeAsO4⋅2H2O and jarosites. S oxidation coupled to Fe3+ reduction occurred at the bottom of the columns, with the formations of FeAsO4⋅2H2O precipitate and S intermediates. The formed FeAsO4⋅2H2O and jarosites at the top and bottom of the columns could adsorb to and coprecipitate with As(III) and As(V), resulting in the transfer of As from solution to solid phases, thus further affecting As speciation transformation. The distribution difference of Fe/S energy substrates could apparently affect Fe/S/As speciation transformation and bacterial depth distribution between the top and bottom of the water columns. These findings are valuable for elucidating As fate and toxicity mediated by microbially driven Fe/S redox in AMD environments.
Keywords: Acidiphilium acidophilum; Acidithiobacillus ferrooxidans; Fe/S redox reactions; Fe/S/As speciation transformation; acid mine drainage.
Copyright © 2022 Zhou, Wang, Liu, Xue, Nie, Liu, Wan, Yang, Shu and Xia.
Conflict of interest statement
The authors declare that the research was conducted in the absence of any commercial or financial relationships that could be construed as a potential conflict of interest.
Figures
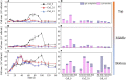
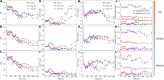
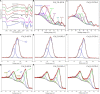
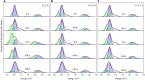
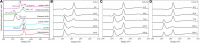
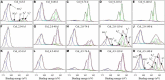
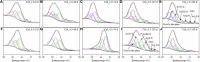
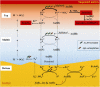
Similar articles
-
The co-culture of Acidithiobacillus ferrooxidans and Acidiphilium acidophilum enhances the growth, iron oxidation, and CO2 fixation.Arch Microbiol. 2011 Dec;193(12):857-66. doi: 10.1007/s00203-011-0723-8. Epub 2011 Jun 21. Arch Microbiol. 2011. PMID: 21691775
-
Effect of ferric ions on the anaerobic bio-dissolution of jarosites by Acidithiobacillus ferrooxidans.Sci Total Environ. 2020 Mar 25;710:136334. doi: 10.1016/j.scitotenv.2019.136334. Epub 2019 Dec 28. Sci Total Environ. 2020. PMID: 32050370
-
Fe/S oxidation-coupled arsenic speciation transformation mediated by AMD enrichment culture under different pH conditions.J Environ Sci (China). 2024 Mar;137:681-700. doi: 10.1016/j.jes.2023.03.008. Epub 2023 Mar 25. J Environ Sci (China). 2024. PMID: 37980051
-
Genetics of metal resistance in acidophilic prokaryotes of acidic mine environments.Indian J Exp Biol. 2004 Jan;42(1):9-25. Indian J Exp Biol. 2004. PMID: 15274476 Review.
-
Genomic insights into microbial iron oxidation and iron uptake strategies in extremely acidic environments.Environ Microbiol. 2012 Jul;14(7):1597-611. doi: 10.1111/j.1462-2920.2011.02626.x. Epub 2011 Nov 3. Environ Microbiol. 2012. PMID: 22050575 Review.
References
-
- Acero P., Ayora C., Torrentó C., Nieto J. M. (2006). The behavior of trace elements during schwertmannite precipitation and subsequent transformation into goethite and jarosite. Geochim. Cosmochim. Acta 70 4130–4139. 10.1016/j.gca.2006.06.1367 - DOI
-
- Burton E. D., Johnston S. G., Planer-Friedrich B. (2013). Coupling of arsenic mobility to sulfur transformations during microbial sulfate reduction in the presence and absence of humic acid. Chem. Geol. 343 12–24. 10.1016/j.chemgeo.2013.02.005 - DOI
LinkOut - more resources
Full Text Sources
Research Materials
Miscellaneous