Seipin forms a flexible cage at lipid droplet formation sites
- PMID: 35210614
- PMCID: PMC8930772
- DOI: 10.1038/s41594-021-00718-y
Seipin forms a flexible cage at lipid droplet formation sites
Abstract
Lipid droplets (LDs) form in the endoplasmic reticulum by phase separation of neutral lipids. This process is facilitated by the seipin protein complex, which consists of a ring of seipin monomers, with a yet unclear function. Here, we report a structure of S. cerevisiae seipin based on cryogenic-electron microscopy and structural modeling data. Seipin forms a decameric, cage-like structure with the lumenal domains forming a stable ring at the cage floor and transmembrane segments forming the cage sides and top. The transmembrane segments interact with adjacent monomers in two distinct, alternating conformations. These conformations result from changes in switch regions, located between the lumenal domains and the transmembrane segments, that are required for seipin function. Our data indicate a model for LD formation in which a closed seipin cage enables triacylglycerol phase separation and subsequently switches to an open conformation to allow LD growth and budding.
© 2022. The Author(s).
Conflict of interest statement
The authors declare no competing interests.
Figures
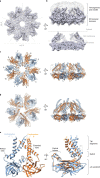
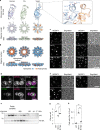
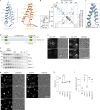
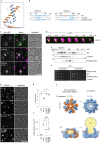
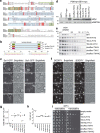
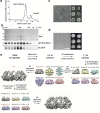
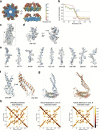
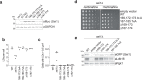
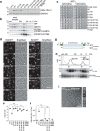
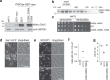
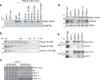
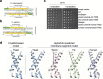
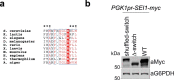
Similar articles
-
Mechanism of lipid droplet formation by the yeast Sei1/Ldb16 Seipin complex.Nat Commun. 2021 Oct 8;12(1):5892. doi: 10.1038/s41467-021-26162-6. Nat Commun. 2021. PMID: 34625558 Free PMC article.
-
Structure and function of lipid droplet assembly complexes.Curr Opin Struct Biol. 2023 Jun;80:102606. doi: 10.1016/j.sbi.2023.102606. Epub 2023 May 5. Curr Opin Struct Biol. 2023. PMID: 37150040 Free PMC article. Review.
-
Human SEIPIN Binds Anionic Phospholipids.Dev Cell. 2018 Oct 22;47(2):248-256.e4. doi: 10.1016/j.devcel.2018.09.010. Epub 2018 Oct 4. Dev Cell. 2018. PMID: 30293840
-
Arabidopsis SEIPIN Proteins Modulate Triacylglycerol Accumulation and Influence Lipid Droplet Proliferation.Plant Cell. 2015 Sep;27(9):2616-36. doi: 10.1105/tpc.15.00588. Epub 2015 Sep 11. Plant Cell. 2015. PMID: 26362606 Free PMC article.
-
A unifying mechanism for seipin-mediated lipid droplet formation.FEBS Lett. 2024 May;598(10):1116-1126. doi: 10.1002/1873-3468.14825. Epub 2024 Feb 13. FEBS Lett. 2024. PMID: 38785192 Free PMC article. Review.
Cited by
-
Seipin transmembrane segments critically function in triglyceride nucleation and lipid droplet budding from the membrane.Elife. 2022 May 18;11:e75808. doi: 10.7554/eLife.75808. Elife. 2022. PMID: 35583926 Free PMC article.
-
Insights Into the Biogenesis and Emerging Functions of Lipid Droplets From Unbiased Molecular Profiling Approaches.Front Cell Dev Biol. 2022 Jun 8;10:901321. doi: 10.3389/fcell.2022.901321. eCollection 2022. Front Cell Dev Biol. 2022. PMID: 35756995 Free PMC article. Review.
-
The evolving landscape of ER-LD contact sites.Front Cell Dev Biol. 2024 Oct 3;12:1483902. doi: 10.3389/fcell.2024.1483902. eCollection 2024. Front Cell Dev Biol. 2024. PMID: 39421023 Free PMC article. Review.
-
Lipid droplets and cellular lipid flux.Nat Cell Biol. 2024 Mar;26(3):331-345. doi: 10.1038/s41556-024-01364-4. Epub 2024 Mar 7. Nat Cell Biol. 2024. PMID: 38454048 Review.
-
Seipin deficiency-induced lipid dysregulation leads to hypomyelination-associated cognitive deficits via compromising oligodendrocyte precursor cell differentiation.Cell Death Dis. 2024 May 21;15(5):350. doi: 10.1038/s41419-024-06737-z. Cell Death Dis. 2024. PMID: 38773070 Free PMC article.
References
-
- Zechner R, Madeo F, Kratky D. Cytosolic lipolysis and lipophagy: two sides of the same coin. Nat. Rev. Mol. Cell Biol. 2017;18:671–684. - PubMed
Publication types
MeSH terms
Substances
Grants and funding
LinkOut - more resources
Full Text Sources
Molecular Biology Databases
Research Materials