Isothermal amplification-assisted diagnostics for COVID-19
- PMID: 35202984
- PMCID: PMC8849862
- DOI: 10.1016/j.bios.2022.114101
Isothermal amplification-assisted diagnostics for COVID-19
Abstract
The scenery of molecular diagnostics for infectious diseases is rapidly evolving to respond to the COVID-19 epidemic. The sensitivity and specificity of diagnostics, along with speed and accuracy, are crucial requirements for effective analytical tools to address the disease spreading around the world. Emerging diagnostic devices combine the latest trends in isothermal amplification methods for nucleic acids with state-of-the-art biosensing systems, intending to bypass roadblocks encountered in the last 2 years of the pandemic. Isothermal nucleic acid amplification is a simple procedure that quickly and efficiently accumulates nucleic acid sequences at a constant temperature, without the need for sophisticated equipment. The integration of isothermal amplification into portable biosensing devices confers high sensitivity and improves screening at the point of need in low-resource settings. This review reports the latest trends reached in this field with the latest examples of isothermal amplification-powered biosensors for detecting SARS-CoV-2, with different configurations, as well as their intrinsic advantages and disadvantages.
Keywords: Biosensors; High throughput; Isothermal amplification; SARS-CoV-2 diagnostics; Sensitive and specific screening.
Copyright © 2022 Elsevier B.V. All rights reserved.
Conflict of interest statement
The authors declare that they have no known competing financial interests or personal relationships that could have appeared to influence the work reported in this paper.
Figures
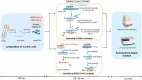
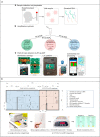
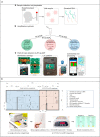
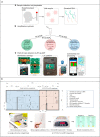
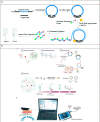
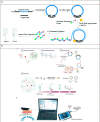
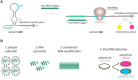
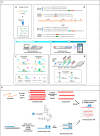
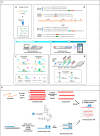
Similar articles
-
High-Surety Isothermal Amplification and Detection of SARS-CoV-2.mSphere. 2021 May 19;6(3):e00911-20. doi: 10.1128/mSphere.00911-20. mSphere. 2021. PMID: 34011690 Free PMC article.
-
SARS-CoV-2 Diagnostics Based on Nucleic Acids Amplification: From Fundamental Concepts to Applications and Beyond.Front Cell Infect Microbiol. 2022 Mar 23;12:799678. doi: 10.3389/fcimb.2022.799678. eCollection 2022. Front Cell Infect Microbiol. 2022. PMID: 35402302 Free PMC article. Review.
-
Toward a next-generation diagnostic tool: A review on emerging isothermal nucleic acid amplification techniques for the detection of SARS-CoV-2 and other infectious viruses.Anal Chim Acta. 2022 May 29;1209:339338. doi: 10.1016/j.aca.2021.339338. Epub 2021 Dec 1. Anal Chim Acta. 2022. PMID: 35569864 Free PMC article. Review.
-
CoLAMP: CRISPR-based one-pot loop-mediated isothermal amplification enables at-home diagnosis of SARS-CoV-2 RNA with nearly eliminated contamination utilizing amplicons depletion strategy.Biosens Bioelectron. 2023 Sep 15;236:115402. doi: 10.1016/j.bios.2023.115402. Epub 2023 May 15. Biosens Bioelectron. 2023. PMID: 37244082 Free PMC article.
-
Colorimetric Reverse Transcription-Loop-Mediated Isothermal Amplification Assay for Rapid Detection of SARS-CoV-2.Am J Trop Med Hyg. 2021 Jun 15;105(2):375-377. doi: 10.4269/ajtmh.21-0150. Am J Trop Med Hyg. 2021. PMID: 34129521 Free PMC article.
Cited by
-
Peptide Nucleic Acid Clamp-Assisted Photothermal Multiplexed Digital PCR for Identifying SARS-CoV-2 Variants of Concern.Adv Sci (Weinh). 2024 Apr;11(13):e2306088. doi: 10.1002/advs.202306088. Epub 2024 Jan 19. Adv Sci (Weinh). 2024. PMID: 38243642 Free PMC article.
-
Paper-Based Biosensors for the Detection of Nucleic Acids from Pathogens.Biosensors (Basel). 2022 Nov 29;12(12):1094. doi: 10.3390/bios12121094. Biosensors (Basel). 2022. PMID: 36551061 Free PMC article. Review.
-
Lateral Flow Assay: A Summary of Recent Progress for Improving Assay Performance.Biosensors (Basel). 2023 Aug 23;13(9):837. doi: 10.3390/bios13090837. Biosensors (Basel). 2023. PMID: 37754072 Free PMC article. Review.
-
Cas12a-assisted RTF-EXPAR for accurate, rapid and simple detection of SARS-CoV-2 RNA.Biosens Bioelectron. 2022 Nov 15;216:114683. doi: 10.1016/j.bios.2022.114683. Epub 2022 Sep 6. Biosens Bioelectron. 2022. PMID: 36088673 Free PMC article.
-
Aptamer-Based Strategies to Address Challenges in COVID-19 Diagnosis and Treatments.Interdiscip Perspect Infect Dis. 2023 Jul 31;2023:9224815. doi: 10.1155/2023/9224815. eCollection 2023. Interdiscip Perspect Infect Dis. 2023. PMID: 37554129 Free PMC article. Review.
References
-
- Augustine R., Hasan A., Das S., Ahmed R., Mori Y., Notomi T., Kevadiya B.D., Thakor A.S. Loop-mediated isothermal amplification (LAMP): a rapid, sensitive, specific, and cost-effective point-of-care test for coronaviruses in the context of COVID-19 pandemic. Biology. 2020;9:182. doi: 10.3390/biology9080182. - DOI - PMC - PubMed
-
- Chakravarthy A., Nandakumar A., George G., Ranganathan S., Umashankar S., Shettigar N., Palakodeti D., Gulyani A., Ramesh A. Engineered RNA biosensors enable ultrasensitive SARS-CoV-2 detection in a simple color and luminescence assay. Life Sci. Alliance. 2021;4 doi: 10.26508/lsa.202101213. - DOI - PMC - PubMed
Publication types
MeSH terms
Substances
LinkOut - more resources
Full Text Sources
Medical
Miscellaneous