The OxyR and SoxR transcriptional regulators are involved in a broad oxidative stress response in Paraburkholderia xenovorans LB400
- PMID: 35184754
- PMCID: PMC8859910
- DOI: 10.1186/s40659-022-00373-7
The OxyR and SoxR transcriptional regulators are involved in a broad oxidative stress response in Paraburkholderia xenovorans LB400
Abstract
Background: Aerobic metabolism generates reactive oxygen species that may cause critical harm to the cell. The aim of this study is the characterization of the stress responses in the model aromatic-degrading bacterium Paraburkholderia xenovorans LB400 to the oxidizing agents paraquat and H2O2.
Methods: Antioxidant genes were identified by bioinformatic methods in the genome of P. xenovorans LB400, and the phylogeny of its OxyR and SoxR transcriptional regulators were studied. Functionality of the transcriptional regulators from strain LB400 was assessed by complementation with LB400 SoxR of null mutant P. aeruginosa ΔsoxR, and the construction of P. xenovorans pIZoxyR that overexpresses OxyR. The effects of oxidizing agents on P. xenovorans were studied measuring bacterial susceptibility, survival and ROS formation after exposure to paraquat and H2O2. The effects of these oxidants on gene expression (qRT-PCR) and the proteome (LC-MS/MS) were quantified.
Results: P. xenovorans LB400 possesses a wide repertoire of genes for the antioxidant defense including the oxyR, ahpC, ahpF, kat, trxB, dpsA and gorA genes, whose orthologous genes are regulated by the transcriptional regulator OxyR in E. coli. The LB400 genome also harbors the soxR, fumC, acnA, sodB, fpr and fldX genes, whose orthologous genes are regulated by the transcriptional regulator SoxR in E. coli. The functionality of the LB400 soxR gene was confirmed by complementation of null mutant P. aeruginosa ΔsoxR. Growth, susceptibility, and ROS formation assays revealed that LB400 cells were more susceptible to paraquat than H2O2. Transcriptional analyses indicated the upregulation of the oxyR, ahpC1, katE and ohrB genes in LB400 cells after exposure to H2O2, whereas the oxyR, fumC, ahpC1, sodB1 and ohrB genes were induced in presence of paraquat. Proteome analysis revealed that paraquat induced the oxidative stress response proteins AhpCF and DpsA, the universal stress protein UspA and the RNA chaperone CspA. Both oxidizing agents induced the Ohr protein, which is involved in organic peroxide resistance. Notably, the overexpression of the LB400 oxyR gene in P. xenovorans significantly decreased the ROS formation and the susceptibility to paraquat, suggesting a broad OxyR-regulated antioxidant response.
Conclusions: This study showed that P. xenovorans LB400 possess a broad range oxidative stress response, which explain the high resistance of this strain to the oxidizing compounds paraquat and H2O2.
Keywords: Hydrogen peroxide; Oxidative stress; OxyR; Paraburkholderia xenovorans; Paraquat; SoxR; Superoxide.
© 2022. The Author(s).
Conflict of interest statement
The authors declare that they have no competing interests.
Figures
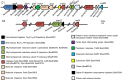
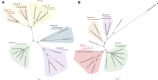
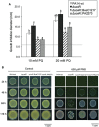
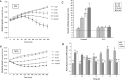
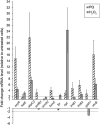
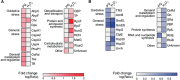
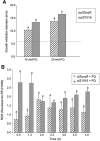
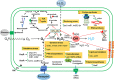
Similar articles
-
The long-chain flavodoxin FldX1 improves the biodegradation of 4-hydroxyphenylacetate and 3-hydroxyphenylacetate and counteracts the oxidative stress associated to aromatic catabolism in Paraburkholderia xenovorans.Biol Res. 2024 Apr 1;57(1):12. doi: 10.1186/s40659-024-00491-4. Biol Res. 2024. PMID: 38561836 Free PMC article.
-
Long-chain flavodoxin FldX1 improves Paraburkholderia xenovorans LB400 tolerance to oxidative stress caused by paraquat and H2O2.PLoS One. 2019 Aug 30;14(8):e0221881. doi: 10.1371/journal.pone.0221881. eCollection 2019. PLoS One. 2019. PMID: 31469877 Free PMC article.
-
Role of the Pseudomonas aeruginosa oxyR-recG operon in oxidative stress defense and DNA repair: OxyR-dependent regulation of katB-ankB, ahpB, and ahpC-ahpF.J Bacteriol. 2000 Aug;182(16):4533-44. doi: 10.1128/JB.182.16.4533-4544.2000. J Bacteriol. 2000. PMID: 10913087 Free PMC article.
-
The OxyR regulon.Antonie Van Leeuwenhoek. 1990 Oct;58(3):157-61. doi: 10.1007/BF00548927. Antonie Van Leeuwenhoek. 1990. PMID: 2256675 Review.
-
OxyR: a regulator of antioxidant genes.J Nutr. 1992 Mar;122(3 Suppl):627-30. doi: 10.1093/jn/122.suppl_3.627. J Nutr. 1992. PMID: 1542022 Review.
Cited by
-
OxyR is required for oxidative stress resistance of the entomopathogenic bacterium Xenorhabdus nematophila and has a minor role during the bacterial interaction with its hosts.Microbiology (Reading). 2024 Jul;170(7):001481. doi: 10.1099/mic.0.001481. Microbiology (Reading). 2024. PMID: 39058385 Free PMC article.
-
Pseudomonas putida as saviour for troubled Synechococcus elongatus in a synthetic co-culture - interaction studies based on a multi-OMICs approach.Commun Biol. 2024 Apr 12;7(1):452. doi: 10.1038/s42003-024-06098-5. Commun Biol. 2024. PMID: 38609451 Free PMC article.
-
The long-chain flavodoxin FldX1 improves the biodegradation of 4-hydroxyphenylacetate and 3-hydroxyphenylacetate and counteracts the oxidative stress associated to aromatic catabolism in Paraburkholderia xenovorans.Biol Res. 2024 Apr 1;57(1):12. doi: 10.1186/s40659-024-00491-4. Biol Res. 2024. PMID: 38561836 Free PMC article.
References
-
- Agulló L, Cámara B, Martínez P, Latorre-Reyes V, Seeger M. Response to (chloro)biphenyls of the PCB-degrader Burkholderia xenovorans LB400 involves stress proteins induced also by heat shock and oxidative stress. FEMS Microbiol Lett. 2007;267:167–175. - PubMed
-
- Altschul SF, Gish W, Miller W, Myers EW, Lipman DJ. Basic local alignment search tool. J Mol Biol. 1990;215:403–410. - PubMed
-
- Apweiler R, Bairoch A, Wu CH. Protein sequence databases. Curr Opin Che Biol. 2004;8:76–80. - PubMed
MeSH terms
Substances
Supplementary concepts
Grants and funding
LinkOut - more resources
Full Text Sources