Respiratory mucosal delivery of next-generation COVID-19 vaccine provides robust protection against both ancestral and variant strains of SARS-CoV-2
- PMID: 35180381
- PMCID: PMC8825346
- DOI: 10.1016/j.cell.2022.02.005
Respiratory mucosal delivery of next-generation COVID-19 vaccine provides robust protection against both ancestral and variant strains of SARS-CoV-2
Abstract
The emerging SARS-CoV-2 variants of concern (VOCs) threaten the effectiveness of current COVID-19 vaccines administered intramuscularly and designed to only target the spike protein. There is a pressing need to develop next-generation vaccine strategies for broader and long-lasting protection. Using adenoviral vectors (Ad) of human and chimpanzee origin, we evaluated Ad-vectored trivalent COVID-19 vaccines expressing spike-1, nucleocapsid, and RdRp antigens in murine models. We show that single-dose intranasal immunization, particularly with chimpanzee Ad-vectored vaccine, is superior to intramuscular immunization in induction of the tripartite protective immunity consisting of local and systemic antibody responses, mucosal tissue-resident memory T cells and mucosal trained innate immunity. We further show that intranasal immunization provides protection against both the ancestral SARS-CoV-2 and two VOC, B.1.1.7 and B.1.351. Our findings indicate that respiratory mucosal delivery of Ad-vectored multivalent vaccine represents an effective next-generation COVID-19 vaccine strategy to induce all-around mucosal immunity against current and future VOC.
Keywords: COVID-19; SARS-CoV-2; T cell immunity; adenoviral vector; animal models; chimpanzee adenoviral vector; human adenoviral vector; humoral immunity; intramuscular immunization; multi-valent vaccine; next-generation vaccines; respiratory mucosal immunity; respiratory mucosal immunization; trained innate immunity; variants of concern.
Copyright © 2022 The Author(s). Published by Elsevier Inc. All rights reserved.
Conflict of interest statement
Declaration of interests B.D.L., F.S., and Z.X. are inventors on a US provisional patent application no. 63/222723, entitled “Novel COVID vaccine and method for delivery.” All other authors declare no competing interests.
Figures
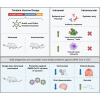
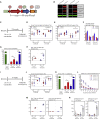
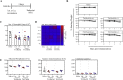
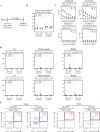
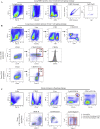
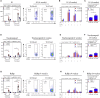
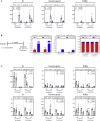
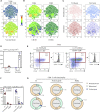
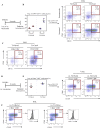
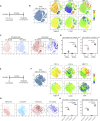
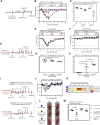
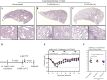
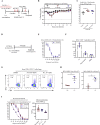
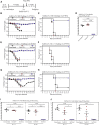
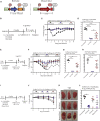
Comment in
-
Route, origin & valence matter: towards sophisticated next-generation vaccines to cope with the COVID-19 pandemic.Signal Transduct Target Ther. 2022 Jun 15;7(1):188. doi: 10.1038/s41392-022-01053-4. Signal Transduct Target Ther. 2022. PMID: 35705533 Free PMC article. No abstract available.
Similar articles
-
Intranasal HD-Ad-FS vaccine induces systemic and airway mucosal immunities against SARS-CoV-2 and systemic immunity against SARS-CoV-2 variants in mice and hamsters.Front Immunol. 2024 Aug 30;15:1430928. doi: 10.3389/fimmu.2024.1430928. eCollection 2024. Front Immunol. 2024. PMID: 39281669 Free PMC article.
-
An Intranasal OMV-Based Vaccine Induces High Mucosal and Systemic Protecting Immunity Against a SARS-CoV-2 Infection.Front Immunol. 2021 Dec 17;12:781280. doi: 10.3389/fimmu.2021.781280. eCollection 2021. Front Immunol. 2021. PMID: 34987509 Free PMC article.
-
Intranasal delivery of a subunit protein vaccine provides protective immunity against JN.1 and XBB-lineage variants.Signal Transduct Target Ther. 2024 Nov 20;9(1):311. doi: 10.1038/s41392-024-02025-6. Signal Transduct Target Ther. 2024. PMID: 39562542 Free PMC article.
-
Intranasal COVID-19 vaccines: From bench to bed.EBioMedicine. 2022 Feb;76:103841. doi: 10.1016/j.ebiom.2022.103841. Epub 2022 Jan 24. EBioMedicine. 2022. PMID: 35085851 Free PMC article. Review.
-
Severe acute respiratory syndrome-coronavirus-2 spike (S) protein based vaccine candidates: State of the art and future prospects.Rev Med Virol. 2021 May;31(3):e2183. doi: 10.1002/rmv.2183. Epub 2020 Oct 15. Rev Med Virol. 2021. PMID: 33594794 Free PMC article. Review.
Cited by
-
Safety, efficacy and immunogenicity of aerosolized Ad5-nCoV COVID-19 vaccine in a non-inferiority randomized controlled trial.NPJ Vaccines. 2024 Oct 31;9(1):209. doi: 10.1038/s41541-024-01003-x. NPJ Vaccines. 2024. PMID: 39482336 Free PMC article.
-
Alcaligenes lipid A functions as a superior mucosal adjuvant to monophosphoryl lipid A via the recruitment and activation of CD11b+ dendritic cells in nasal tissue.Int Immunol. 2024 Jan 29;36(1):33-43. doi: 10.1093/intimm/dxad045. Int Immunol. 2024. PMID: 38006376 Free PMC article.
-
SARS-CoV-2: pathogenesis, therapeutics, variants, and vaccines.Front Microbiol. 2024 Jun 13;15:1334152. doi: 10.3389/fmicb.2024.1334152. eCollection 2024. Front Microbiol. 2024. PMID: 38939189 Free PMC article. Review.
-
The protective immunity induced by intranasally inoculated serotype 63 chimpanzee adenovirus vector expressing human respiratory syncytial virus prefusion fusion glycoprotein in BALB/c mice.Front Microbiol. 2022 Nov 18;13:1041338. doi: 10.3389/fmicb.2022.1041338. eCollection 2022. Front Microbiol. 2022. PMID: 36466668 Free PMC article.
-
Editorial: Adaptive immunity to respiratory pathogens.Front Immunol. 2023 Mar 6;14:1174178. doi: 10.3389/fimmu.2023.1174178. eCollection 2023. Front Immunol. 2023. PMID: 36949940 Free PMC article. No abstract available.
References
Publication types
MeSH terms
Substances
Supplementary concepts
LinkOut - more resources
Full Text Sources
Other Literature Sources
Medical
Molecular Biology Databases
Research Materials
Miscellaneous