Age-Dependent Reduction in Asthmatic Pathology through Reprogramming of Postviral Inflammatory Responses
- PMID: 35173037
- PMCID: PMC8917060
- DOI: 10.4049/jimmunol.2101094
Age-Dependent Reduction in Asthmatic Pathology through Reprogramming of Postviral Inflammatory Responses
Abstract
Asthma is a chronic disease of childhood, but for unknown reasons, disease activity sometimes subsides as children mature. In this study, we present clinical and animal model evidence suggesting that the age dependency of childhood asthma stems from an evolving host response to respiratory viral infection. Using clinical data, we show that societal suppression of respiratory virus transmission during coronavirus disease 2019 lockdown disrupted the traditional age gradient in pediatric asthma exacerbations, connecting the phenomenon of asthma remission to virus exposure. In mice, we show that asthmatic lung pathology triggered by Sendai virus (SeV) or influenza A virus is highly age-sensitive: robust in juvenile mice (4-6 wk old) but attenuated in mature mice (>3 mo old). Interestingly, allergen induction of the same asthmatic traits was less dependent on chronological age than viruses. Age-specific responses to SeV included a juvenile bias toward type 2 airway inflammation that emerged early in infection, whereas mature mice exhibited a more restricted bronchiolar distribution of infection that produced a distinct type 2 low inflammatory cytokine profile. In the basal state, aging produced changes to lung leukocyte burden, including the number and transcriptional landscape of alveolar macrophages (AMs). Importantly, depleting AMs in mature mice restored post-SeV pathology to juvenile levels. Thus, aging influences chronic outcomes of respiratory viral infection through regulation of the AM compartment and type 2 inflammatory responses to viruses. Our data provide insight into how asthma remission might develop in children.
Copyright © 2022 by The American Association of Immunologists, Inc.
Conflict of interest statement
DISCLOSURES
The authors report no conflict of interest.
Figures
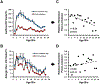
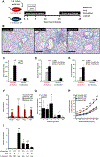
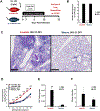
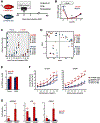
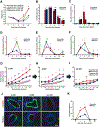
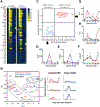
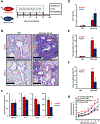
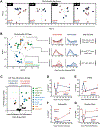
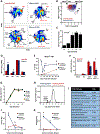
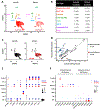
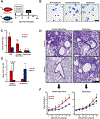
Similar articles
-
BMAL1 links the circadian clock to viral airway pathology and asthma phenotypes.Mucosal Immunol. 2018 Jan;11(1):97-111. doi: 10.1038/mi.2017.24. Epub 2017 Apr 12. Mucosal Immunol. 2018. PMID: 28401936 Free PMC article.
-
Initial Influenza Virus Replication Can Be Limited in Allergic Asthma Through Rapid Induction of Type III Interferons in Respiratory Epithelium.Front Immunol. 2018 May 17;9:986. doi: 10.3389/fimmu.2018.00986. eCollection 2018. Front Immunol. 2018. PMID: 29867963 Free PMC article.
-
Distinct Chronic Post-Viral Lung Diseases upon Infection with Influenza or Parainfluenza Viruses Differentially Impact Superinfection Outcome.Am J Pathol. 2020 Mar;190(3):543-553. doi: 10.1016/j.ajpath.2019.11.003. Epub 2019 Dec 19. Am J Pathol. 2020. PMID: 31866346 Free PMC article.
-
Coronavirus disease 2019 and asthma, allergic rhinitis: molecular mechanisms and host-environmental interactions.Curr Opin Allergy Clin Immunol. 2021 Feb 1;21(1):1-7. doi: 10.1097/ACI.0000000000000699. Curr Opin Allergy Clin Immunol. 2021. PMID: 33186186 Review.
-
Influenza in Asthmatics: For Better or for Worse?Front Immunol. 2018 Aug 10;9:1843. doi: 10.3389/fimmu.2018.01843. eCollection 2018. Front Immunol. 2018. PMID: 30147697 Free PMC article. Review.
Cited by
-
The breath volatilome is shaped by the gut microbiota.medRxiv [Preprint]. 2024 Aug 8:2024.08.02.24311413. doi: 10.1101/2024.08.02.24311413. medRxiv. 2024. PMID: 39132488 Free PMC article. Preprint.
-
Effect of the COVID-19 Lockdown on Asthma Biological Rhythms.J Biol Rhythms. 2022 Apr;37(2):152-163. doi: 10.1177/07487304221081730. Epub 2022 Mar 23. J Biol Rhythms. 2022. PMID: 35319293 Free PMC article.
-
Age-dependent rebound in asthma exacerbations after COVID-19 lockdown.J Allergy Clin Immunol Glob. 2022 Nov;1(4):314-318. doi: 10.1016/j.jacig.2022.06.001. Epub 2022 Aug 10. J Allergy Clin Immunol Glob. 2022. PMID: 36377231 Free PMC article.
-
Multi-organ phenotypes in mice lacking latent TGFβ binding protein 2 (LTBP2).Dev Dyn. 2024 Feb;253(2):233-254. doi: 10.1002/dvdy.651. Epub 2023 Sep 9. Dev Dyn. 2024. PMID: 37688792 Free PMC article. Review.
References
-
- Mims JW 2015. Asthma: definitions and pathophysiology. Int Forum Allergy Rhinol 5 Suppl 1: S2–6. - PubMed
-
- Yunginger JW, Reed CE, O’Connell EJ, Melton LJ 3rd, O’Fallon WM, and Silverstein MD. 1992. A community-based study of the epidemiology of asthma. Incidence rates, 1964–1983. The American review of respiratory disease 146: 888–894. - PubMed
-
- Wenzel SE 2012. Asthma phenotypes: the evolution from clinical to molecular approaches. Nature medicine 18: 716–725. - PubMed