Dissecting the Mechanism of Action of Spiperone-A Candidate for Drug Repurposing for Colorectal Cancer
- PMID: 35159043
- PMCID: PMC8834219
- DOI: 10.3390/cancers14030776
Dissecting the Mechanism of Action of Spiperone-A Candidate for Drug Repurposing for Colorectal Cancer
Abstract
Approximately 50% of colorectal cancer (CRC) patients still die from recurrence and metastatic disease, highlighting the need for novel therapeutic strategies. Drug repurposing is attracting increasing attention because, compared to traditional de novo drug discovery processes, it may reduce drug development periods and costs. Epidemiological and preclinical evidence support the antitumor activity of antipsychotic drugs. Herein, we dissect the mechanism of action of the typical antipsychotic spiperone in CRC. Spiperone can reduce the clonogenic potential of stem-like CRC cells (CRC-SCs) and induce cell cycle arrest and apoptosis, in both differentiated and CRC-SCs, at clinically relevant concentrations whose toxicity is negligible for non-neoplastic cells. Analysis of intracellular Ca2+ kinetics upon spiperone treatment revealed a massive phospholipase C (PLC)-dependent endoplasmic reticulum (ER) Ca2+ release, resulting in ER Ca2+ homeostasis disruption. RNA sequencing revealed unfolded protein response (UPR) activation, ER stress, and induction of apoptosis, along with IRE1-dependent decay of mRNA (RIDD) activation. Lipidomic analysis showed a significant alteration of lipid profile and, in particular, of sphingolipids. Damage to the Golgi apparatus was also observed. Our data suggest that spiperone can represent an effective drug in the treatment of CRC, and that ER stress induction, along with lipid metabolism alteration, represents effective druggable pathways in CRC.
Keywords: Golgi; cancer stem cells; colorectal cancer; endoplasmic reticulum stress; intracellular calcium; lipid metabolism; mitochondria; phospholipase C; psychotropic drugs; repurposing.
Conflict of interest statement
The authors declare no conflict of interest. The funders had no role in the design of the study; in the collection, analyses, or interpretation of data; in the writing of the manuscript, or in the decision to publish the results.
Figures
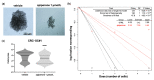
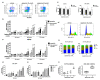
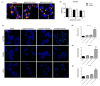
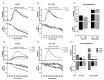
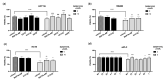
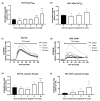
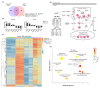
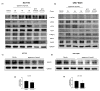
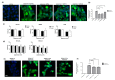
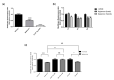
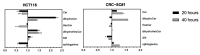
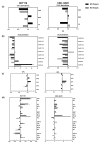
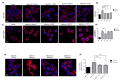
Similar articles
-
Functional Coupling between the Unfolded Protein Response and Endoplasmic Reticulum/Golgi Ca2+-ATPases Promotes Stress Tolerance, Cell Wall Biosynthesis, and Virulence of Aspergillus fumigatus.mBio. 2020 Jun 2;11(3):e01060-20. doi: 10.1128/mBio.01060-20. mBio. 2020. PMID: 32487759 Free PMC article.
-
Knockdown of CEMIP suppresses proliferation and induces apoptosis in colorectal cancer cells: downregulation of GRP78 and attenuation of unfolded protein response.Biochem Cell Biol. 2018 Jun;96(3):332-341. doi: 10.1139/bcb-2017-0151. Epub 2017 Oct 12. Biochem Cell Biol. 2018. PMID: 29024602
-
PERK activation by CCT020312 chemosensitizes colorectal cancer through inducing apoptosis regulated by ER stress.Biochem Biophys Res Commun. 2021 Jun 11;557:316-322. doi: 10.1016/j.bbrc.2021.03.041. Epub 2021 Apr 21. Biochem Biophys Res Commun. 2021. PMID: 33894420
-
Getting RIDD of RNA: IRE1 in cell fate regulation.Trends Biochem Sci. 2014 May;39(5):245-54. doi: 10.1016/j.tibs.2014.02.008. Epub 2014 Mar 20. Trends Biochem Sci. 2014. PMID: 24657016 Review.
-
ER stress-induced cell death mechanisms.Biochim Biophys Acta. 2013 Dec;1833(12):3460-3470. doi: 10.1016/j.bbamcr.2013.06.028. Epub 2013 Jul 10. Biochim Biophys Acta. 2013. PMID: 23850759 Free PMC article. Review.
Cited by
-
Targeting lysine-specific demethylase 1 (KDM1A/LSD1) impairs colorectal cancer tumorigenesis by affecting cancer cells stemness, motility, and differentiation.Cell Death Discov. 2023 Jun 29;9(1):201. doi: 10.1038/s41420-023-01502-1. Cell Death Discov. 2023. PMID: 37385999 Free PMC article.
-
Current trends and future prospects of drug repositioning in gastrointestinal oncology.Front Pharmacol. 2024 Jan 4;14:1329244. doi: 10.3389/fphar.2023.1329244. eCollection 2023. Front Pharmacol. 2024. PMID: 38239190 Free PMC article. Review.
-
Chewing the fat: How lipidomics is changing our understanding of human health and disease in 2022.Anal Sci Adv. 2023 May 10;4(3-4):104-131. doi: 10.1002/ansa.202300009. eCollection 2023 May. Anal Sci Adv. 2023. PMID: 38715925 Free PMC article. Review.
References
Grants and funding
LinkOut - more resources
Full Text Sources
Molecular Biology Databases
Miscellaneous