Ppt1-deficiency dysregulates lysosomal Ca++ homeostasis contributing to pathogenesis in a mouse model of CLN1 disease
- PMID: 35150145
- PMCID: PMC9090967
- DOI: 10.1002/jimd.12485
Ppt1-deficiency dysregulates lysosomal Ca++ homeostasis contributing to pathogenesis in a mouse model of CLN1 disease
Abstract
Inactivating mutations in the PPT1 gene encoding palmitoyl-protein thioesterase-1 (PPT1) underlie the CLN1 disease, a devastating neurodegenerative lysosomal storage disorder. The mechanism of pathogenesis underlying CLN1 disease has remained elusive. PPT1 is a lysosomal enzyme, which catalyzes the removal of palmitate from S-palmitoylated proteins (constituents of ceroid lipofuscin) facilitating their degradation and clearance by lysosomal hydrolases. Thus, it has been proposed that Ppt1-deficiency leads to lysosomal accumulation of ceroid lipofuscin leading to CLN1 disease. While S-palmitoylation is catalyzed by palmitoyl acyltransferases (called ZDHHCs), palmitoyl-protein thioesterases (PPTs) depalmitoylate these proteins. We sought to determine the mechanism by which Ppt1-deficiency may impair lysosomal degradative function leading to infantile neuronal ceroid lipofuscinosis pathogenesis. Here, we report that in Ppt1-/- mice, which mimic CLN1 disease, low level of inositol 3-phosphate receptor-1 (IP3R1) that mediates Ca++ transport from the endoplasmic reticulum to the lysosome dysregulated lysosomal Ca++ homeostasis. Intriguingly, the transcription factor nuclear factor of activated T-cells, cytoplasmic 4 (NFATC4), which regulates IP3R1-expression, required S-palmitoylation for trafficking from the cytoplasm to the nucleus. We identified two palmitoyl acyltransferases, ZDHHC4 and ZDHHC8, which catalyzed S-palmitoylation of NFATC4. Notably, in Ppt1-/- mice, reduced ZDHHC4 and ZDHHC8 levels markedly lowered S-palmitoylated NFATC4 (active) in the nucleus, which inhibited IP3R1-expression, thereby dysregulating lysosomal Ca++ homeostasis. Consequently, Ca++ -dependent lysosomal enzyme activities were markedly suppressed. Impaired lysosomal degradative function impaired autophagy, which caused lysosomal storage of undigested cargo. Importantly, IP3R1-overexpression in Ppt1-/- mouse fibroblasts ameliorated this defect. Our results reveal a previously unrecognized role of Ppt1 in regulating lysosomal Ca++ homeostasis and suggest that this defect contributes to pathogenesis of CLN1 disease.
Keywords: Batten disease; S-palmitoylation; infantile neuronal ceroid lipofuscinosis; lysosomal storage disease; neurodegeneration; neuronal ceroid lipofuscinosis; palmitoyl-protein thioesterase-1.
Published 2022. This article is a U.S. Government work and is in the public domain in the USA.
Conflict of interest statement
Figures
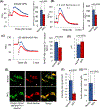
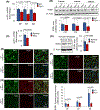
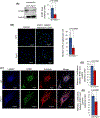
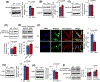
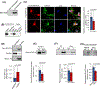
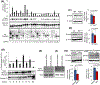
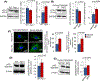
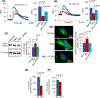
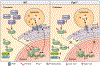
Similar articles
-
Cln1-mutations suppress Rab7-RILP interaction and impair autophagy contributing to neuropathology in a mouse model of infantile neuronal ceroid lipofuscinosis.J Inherit Metab Dis. 2020 Sep;43(5):1082-1101. doi: 10.1002/jimd.12242. Epub 2020 Apr 27. J Inherit Metab Dis. 2020. PMID: 32279353 Free PMC article.
-
Cln3-mutations underlying juvenile neuronal ceroid lipofuscinosis cause significantly reduced levels of Palmitoyl-protein thioesterases-1 (Ppt1)-protein and Ppt1-enzyme activity in the lysosome.J Inherit Metab Dis. 2019 Sep;42(5):944-954. doi: 10.1002/jimd.12106. Epub 2019 May 14. J Inherit Metab Dis. 2019. PMID: 31025705 Free PMC article.
-
Disruption of lysosomal nutrient sensing scaffold contributes to pathogenesis of a fatal neurodegenerative lysosomal storage disease.J Biol Chem. 2024 Feb;300(2):105641. doi: 10.1016/j.jbc.2024.105641. Epub 2024 Jan 9. J Biol Chem. 2024. PMID: 38211816 Free PMC article.
-
Pathogenesis and therapies for infantile neuronal ceroid lipofuscinosis (infantile CLN1 disease).Biochim Biophys Acta. 2013 Nov;1832(11):1906-9. doi: 10.1016/j.bbadis.2013.05.026. Epub 2013 Jun 6. Biochim Biophys Acta. 2013. PMID: 23747979 Free PMC article. Review.
-
Cell biology and function of neuronal ceroid lipofuscinosis-related proteins.Biochim Biophys Acta. 2013 Nov;1832(11):1866-81. doi: 10.1016/j.bbadis.2013.01.019. Epub 2013 Feb 9. Biochim Biophys Acta. 2013. PMID: 23402926 Review.
Cited by
-
Palmitoylation by ZDHHC4 inhibits TRPV1-mediated nociception.EMBO Rep. 2025 Jan;26(1):101-121. doi: 10.1038/s44319-024-00317-0. Epub 2024 Nov 11. EMBO Rep. 2025. PMID: 39528731 Free PMC article.
-
Proteomic predictors of individualized nutrient-specific insulin secretion in health and disease.Cell Metab. 2024 Jul 2;36(7):1619-1633.e5. doi: 10.1016/j.cmet.2024.06.001. Cell Metab. 2024. PMID: 38959864 Free PMC article.
-
Lysosomal lipid peroxidation regulates tumor immunity.J Clin Invest. 2023 Apr 17;133(8):e164596. doi: 10.1172/JCI164596. J Clin Invest. 2023. PMID: 36795483 Free PMC article.
-
Cellular miR-6741-5p as a Prognostic Biomarker Predicting Length of Hospital Stay among COVID-19 Patients.Viruses. 2022 Nov 30;14(12):2681. doi: 10.3390/v14122681. Viruses. 2022. PMID: 36560686 Free PMC article.
References
-
- De Duve C & Wattiaux R Functions of lysosomes. Annu. Rev. Physiol 1966; 28: 435–492. - PubMed
-
- Parenti G, Andria G & Ballabio A Lysosomal storage diseases: from pathophysiology to therapy. Annu Rev Med 2015; 66: 471–486. - PubMed
-
- Futerman AH & van Meer G The cell biology of lysosomal storage disorders. Nat Rev Mol Cell Biol 2004; 5: 554–565 - PubMed
Publication types
MeSH terms
Substances
Grants and funding
LinkOut - more resources
Full Text Sources
Molecular Biology Databases
Research Materials
Miscellaneous