Strategies for Heterologous Expression, Synthesis, and Purification of Animal Venom Toxins
- PMID: 35127675
- PMCID: PMC8811309
- DOI: 10.3389/fbioe.2021.811905
Strategies for Heterologous Expression, Synthesis, and Purification of Animal Venom Toxins
Abstract
Animal venoms are complex mixtures containing peptides and proteins known as toxins, which are responsible for the deleterious effect of envenomations. Across the animal Kingdom, toxin diversity is enormous, and the ability to understand the biochemical mechanisms governing toxicity is not only relevant for the development of better envenomation therapies, but also for exploiting toxin bioactivities for therapeutic or biotechnological purposes. Most of toxinology research has relied on obtaining the toxins from crude venoms; however, some toxins are difficult to obtain because the venomous animal is endangered, does not thrive in captivity, produces only a small amount of venom, is difficult to milk, or only produces low amounts of the toxin of interest. Heterologous expression of toxins enables the production of sufficient amounts to unlock the biotechnological potential of these bioactive proteins. Moreover, heterologous expression ensures homogeneity, avoids cross-contamination with other venom components, and circumvents the use of crude venom. Heterologous expression is also not only restricted to natural toxins, but allows for the design of toxins with special properties or can take advantage of the increasing amount of transcriptomics and genomics data, enabling the expression of dormant toxin genes. The main challenge when producing toxins is obtaining properly folded proteins with a correct disulfide pattern that ensures the activity of the toxin of interest. This review presents the strategies that can be used to express toxins in bacteria, yeast, insect cells, or mammalian cells, as well as synthetic approaches that do not involve cells, such as cell-free biosynthesis and peptide synthesis. This is accompanied by an overview of the main advantages and drawbacks of these different systems for producing toxins, as well as a discussion of the biosafety considerations that need to be made when working with highly bioactive proteins.
Keywords: animal toxins; bioinsecticide; heterologous expression; neurotoxin; recombinant protein expression; recombinant toxins; toxin-inspired drug; venom.
Copyright © 2022 Rivera-de-Torre, Rimbault, Jenkins, Sørensen, Damsbo, Saez, Duhoo, Hackney, Ellgaard and Laustsen.
Conflict of interest statement
The authors declare that the research was conducted in the absence of any commercial or financial relationships that could be construed as a potential conflict of interest.
Figures
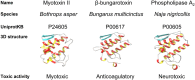
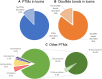
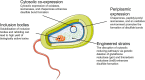
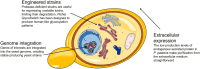
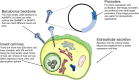
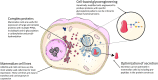

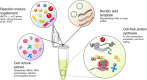
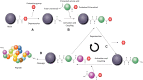
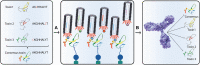
Similar articles
-
[Ants: a chemical library of anticancer molecules].Biol Aujourdhui. 2016;210(2):119-25. doi: 10.1051/jbio/2016021. Epub 2016 Sep 30. Biol Aujourdhui. 2016. PMID: 27687602 Review. French.
-
Microbial production of toxins from the scorpion venom: properties and applications.Appl Microbiol Biotechnol. 2018 Aug;102(15):6319-6331. doi: 10.1007/s00253-018-9122-2. Epub 2018 Jun 1. Appl Microbiol Biotechnol. 2018. PMID: 29858954 Review.
-
Molecular cloning and functional characterization of two isoforms of dermonecrotic toxin from Loxosceles intermedia (brown spider) venom gland.Biochimie. 2006 Sep;88(9):1241-53. doi: 10.1016/j.biochi.2006.02.008. Epub 2006 Mar 23. Biochimie. 2006. PMID: 16581177
-
Production, composition, and mode of action of the painful defensive venom produced by a limacodid caterpillar, Doratifera vulnerans.Proc Natl Acad Sci U S A. 2021 May 4;118(18):e2023815118. doi: 10.1073/pnas.2023815118. Proc Natl Acad Sci U S A. 2021. PMID: 33893140 Free PMC article.
-
The medical threat of mamba envenoming in sub-Saharan Africa revealed by genus-wide analysis of venom composition, toxicity and antivenomics profiling of available antivenoms.J Proteomics. 2018 Feb 10;172:173-189. doi: 10.1016/j.jprot.2017.08.016. Epub 2017 Aug 24. J Proteomics. 2018. PMID: 28843532
Cited by
-
Neurotoxin-Derived Optical Probes for Biological and Medical Imaging.Mol Imaging Biol. 2023 Oct;25(5):799-814. doi: 10.1007/s11307-023-01838-1. Epub 2023 Jul 19. Mol Imaging Biol. 2023. PMID: 37468801 Free PMC article. Review.
-
Exploring the Diversity and Function of Serine Proteases in Toxicofera Reptile Venoms: A Comprehensive Overview.Toxins (Basel). 2024 Oct 3;16(10):428. doi: 10.3390/toxins16100428. Toxins (Basel). 2024. PMID: 39453204 Free PMC article. Review.
-
Venom biotechnology: casting light on nature's deadliest weapons using synthetic biology.Front Bioeng Biotechnol. 2023 May 3;11:1166601. doi: 10.3389/fbioe.2023.1166601. eCollection 2023. Front Bioeng Biotechnol. 2023. PMID: 37207126 Free PMC article. Review.
-
The Toxin Diversity, Cytotoxicity, and Enzymatic Activity of Cape Cobra (Naja nivea) Venom.Toxins (Basel). 2024 Oct 11;16(10):438. doi: 10.3390/toxins16100438. Toxins (Basel). 2024. PMID: 39453214 Free PMC article.
-
Venom Ex Machina? Exploring the Potential of Cell-Free Protein Production for Venom Biodiscovery.Int J Mol Sci. 2024 Jul 29;25(15):8286. doi: 10.3390/ijms25158286. Int J Mol Sci. 2024. PMID: 39125859 Free PMC article.
References
-
- Alegre-Cebollada J., Clementi G., Cunietti M., Porres C., Oñaderra M., Gavilanes J. G., et al. (2007). Silent Mutations at the 5′-end of the cDNA of Actinoporins from the Sea Anemone Stichodactyla Helianthus Allow Their Heterologous Overproduction in Escherichia coli . J. Biotechnol. 127, 211–221. 10.1016/j.jbiotec.2006.07.006 - DOI - PubMed
Publication types
Grants and funding
LinkOut - more resources
Full Text Sources