Leveraging interacting signaling pathways to robustly improve the quality and yield of human pluripotent stem cell-derived hepatoblasts and hepatocytes
- PMID: 35120625
- PMCID: PMC9039749
- DOI: 10.1016/j.stemcr.2022.01.003
Leveraging interacting signaling pathways to robustly improve the quality and yield of human pluripotent stem cell-derived hepatoblasts and hepatocytes
Abstract
Pluripotent stem cell (PSC)-derived hepatocyte-like cells (HLCs) have shown great potential as an alternative to primary human hepatocytes (PHHs) for in vitro modeling. Several differentiation protocols have been described to direct PSCs toward the hepatic fate. Here, by leveraging recent knowledge of the signaling pathways involved in liver development, we describe a robust, scalable protocol that allowed us to consistently generate high-quality bipotent human hepatoblasts and HLCs from both embryonic stem cells and induced PSC (iPSCs). Although not yet fully mature, such HLCs were more similar to adult PHHs than were cells obtained with previously described protocols, showing good potential as a physiologically representative alternative to PHHs for in vitro modeling. PSC-derived hepatoblasts effectively generated with this protocol could differentiate into mature hepatocytes and cholangiocytes within syngeneic liver organoids, thus opening the way for representative human 3D in vitro modeling of liver development and pathophysiology.
Keywords: differentiation; disease modeling; drug discovery; hepatoblasts; hepatocyte-like cells; human; induced pluripotent stem cells; liver development; liver organoids; pluripotent stem cells.
Copyright © 2022 The Authors. Published by Elsevier Inc. All rights reserved.
Figures
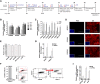
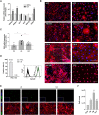
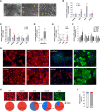
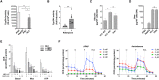
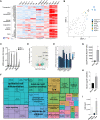
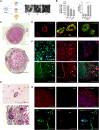
Similar articles
-
Hepatocyte-like cells derived from human induced pluripotent stem cells using small molecules: implications of a transcriptomic study.Stem Cell Res Ther. 2020 Sep 11;11(1):393. doi: 10.1186/s13287-020-01914-1. Stem Cell Res Ther. 2020. PMID: 32917265 Free PMC article.
-
Generation of Complex Syngeneic Liver Organoids from Induced Pluripotent Stem Cells to Model Human Liver Pathophysiology.Curr Protoc. 2022 Mar;2(3):e389. doi: 10.1002/cpz1.389. Curr Protoc. 2022. PMID: 35263041
-
Analysis of differentially expressed genes among human hair follicle-derived iPSCs, induced hepatocyte-like cells, and primary hepatocytes.Stem Cell Res Ther. 2018 Aug 9;9(1):211. doi: 10.1186/s13287-018-0940-z. Stem Cell Res Ther. 2018. PMID: 30092828 Free PMC article.
-
An Extracellular Matrix-based Culture System for Generation of Human Pluripotent Stem Cell Derived-hepatocytes.Curr Stem Cell Res Ther. 2021;16(7):888-896. doi: 10.2174/1574888X16666201228144834. Curr Stem Cell Res Ther. 2021. PMID: 33371861 Review.
-
Pluripotent Stem Cell-Derived Hepatocyte-like Cells: Induction Methods and Applications.Int J Mol Sci. 2023 Jul 18;24(14):11592. doi: 10.3390/ijms241411592. Int J Mol Sci. 2023. PMID: 37511351 Free PMC article. Review.
Cited by
-
iPSC-derived cells for whole liver bioengineering.Front Bioeng Biotechnol. 2024 Feb 7;12:1338762. doi: 10.3389/fbioe.2024.1338762. eCollection 2024. Front Bioeng Biotechnol. 2024. PMID: 38384436 Free PMC article. Review.
-
Chemical approaches targeting the hurdles of hepatocyte transplantation: mechanisms, applications, and advances.Front Cell Dev Biol. 2024 Oct 31;12:1480226. doi: 10.3389/fcell.2024.1480226. eCollection 2024. Front Cell Dev Biol. 2024. PMID: 39544361 Free PMC article. Review.
-
Modeling human liver organ development and diseases with pluripotent stem cell-derived organoids.Front Cell Dev Biol. 2023 Feb 15;11:1133534. doi: 10.3389/fcell.2023.1133534. eCollection 2023. Front Cell Dev Biol. 2023. PMID: 36875751 Free PMC article. Review.
-
Autologous humanized mouse models of iPSC-derived tumors enable characterization and modulation of cancer-immune cell interactions.Cell Rep Methods. 2022 Jan 14;2(1):100153. doi: 10.1016/j.crmeth.2021.100153. eCollection 2022 Jan 24. Cell Rep Methods. 2022. PMID: 35474871 Free PMC article.
-
GATA6 is predicted to regulate DNA methylation in an in vitro model of human hepatocyte differentiation.Commun Biol. 2022 May 4;5(1):414. doi: 10.1038/s42003-022-03365-1. Commun Biol. 2022. PMID: 35508708 Free PMC article.
References
-
- Baxter M., Withey S., Harrison S., Segeritz C.-P., Zhang F., Atkinson-Dell R., Rowe C., Gerrard D.T., Sison-Young R., Jenkins R., et al. Phenotypic and functional analyses show stem cell-derived hepatocyte-like cells better mimic fetal rather than adult hepatocytes. J. Hepatol. 2015;62:581–589. - PMC - PubMed
-
- Berger D.R., Ware B.R., Davidson M.D., Allsup S.R., Khetani S.R. Enhancing the functional maturity of induced pluripotent stem cell-derived human hepatocytes by controlled presentation of cell-cell interactions in vitro. Hepatology. 2015;61:1370–1381. - PubMed
-
- den Braver-Sewradj S.P., den Braver M.W., Vermeulen N.P.E., Commandeur J.N.M., Richert L., Vos J.C. Inter-donor variability of phase I/phase II metabolism of three reference drugs in cryopreserved primary human hepatocytes in suspension and monolayer. Toxicol. Vitro. 2016;33:71–79. - PubMed
Publication types
MeSH terms
Grants and funding
LinkOut - more resources
Full Text Sources
Molecular Biology Databases