A Liquid State Perspective on Dynamics of Chromatin Compartments
- PMID: 35096966
- PMCID: PMC8793688
- DOI: 10.3389/fmolb.2021.781981
A Liquid State Perspective on Dynamics of Chromatin Compartments
Abstract
The interior of the eukaryotic cell nucleus has a crowded and heterogeneous environment packed with chromatin polymers, regulatory proteins, and RNA molecules. Chromatin polymer, assisted by epigenetic modifications, protein and RNA binders, forms multi-scale compartments which help regulate genes in response to cellular signals. Furthermore, chromatin compartments are dynamic and tend to evolve in size and composition in ways that are not fully understood. The latest super-resolution imaging experiments have revealed a much more dynamic and stochastic nature of chromatin compartments than was appreciated before. An emerging mechanism explaining chromatin compartmentalization dynamics is the phase separation of protein and nucleic acids into membraneless liquid condensates. Consequently, concepts and ideas from soft matter and polymer systems have been rapidly entering the lexicon of cell biology. In this respect, the role of computational models is crucial for establishing a rigorous and quantitative foundation for the new concepts and disentangling the complex interplay of forces that contribute to the emergent patterns of chromatin dynamics and organization. Several multi-scale models have emerged to address various aspects of chromatin dynamics, ranging from equilibrium polymer simulations, hybrid non-equilibrium simulations coupling protein binding and chromatin folding, and mesoscopic field-theoretic models. Here, we review these emerging theoretical paradigms and computational models with a particular focus on chromatin's phase separation and liquid-like properties as a basis for nuclear organization and dynamics.
Keywords: chromatin; euchromatin; heterochromatin; imaging; lamin; liquid-liquid phase separation; mesoscale; nuclear organization.
Copyright © 2022 Laghmach, Di Pierro and Potoyan.
Conflict of interest statement
The authors declare that the research was conducted in the absence of any commercial or financial relationships that could be construed as a potential conflict of interest.
Figures
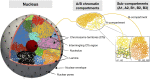
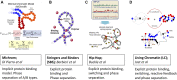
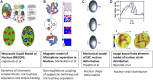
Similar articles
-
Macromolecular crowding: chemistry and physics meet biology (Ascona, Switzerland, 10-14 June 2012).Phys Biol. 2013 Aug;10(4):040301. doi: 10.1088/1478-3975/10/4/040301. Epub 2013 Aug 2. Phys Biol. 2013. PMID: 23912807
-
The interplay of chromatin phase separation and lamina interactions in nuclear organization.Biophys J. 2021 Nov 16;120(22):5005-5017. doi: 10.1016/j.bpj.2021.10.012. Epub 2021 Oct 13. Biophys J. 2021. PMID: 34653387 Free PMC article.
-
Four-Dimensional Mesoscale Liquid Model of Nucleus Resolves Chromatin's Radial Organization.PRX Life. 2024 Jan-Mar;2(1):013006. doi: 10.1103/PRXLife.2.013006. Epub 2024 Jan 30. PRX Life. 2024. PMID: 38601142 Free PMC article.
-
Interplay of dynamic genome organization and biomolecular condensates.Curr Opin Cell Biol. 2023 Dec;85:102252. doi: 10.1016/j.ceb.2023.102252. Epub 2023 Oct 6. Curr Opin Cell Biol. 2023. PMID: 37806293 Review.
-
Evidence for and against Liquid-Liquid Phase Separation in the Nucleus.Noncoding RNA. 2019 Nov 1;5(4):50. doi: 10.3390/ncrna5040050. Noncoding RNA. 2019. PMID: 31683819 Free PMC article. Review.
Cited by
-
From the Catastrophic Objective Irreproducibility of Cancer Research and Unavoidable Failures of Molecular Targeted Therapies to the Sparkling Hope of Supramolecular Targeted Strategies.Int J Mol Sci. 2023 Feb 1;24(3):2796. doi: 10.3390/ijms24032796. Int J Mol Sci. 2023. PMID: 36769134 Free PMC article. Review.
-
DNA choreography: correlating mobility and organization of DNA across different resolutions from loops to chromosomes.Histochem Cell Biol. 2024 Jul;162(1-2):109-131. doi: 10.1007/s00418-024-02285-x. Epub 2024 May 17. Histochem Cell Biol. 2024. PMID: 38758428 Free PMC article.
-
Phase Separation-Mediated Chromatin Organization and Dynamics: From Imaging-Based Quantitative Characterizations to Functional Implications.Int J Mol Sci. 2022 Jul 21;23(14):8039. doi: 10.3390/ijms23148039. Int J Mol Sci. 2022. PMID: 35887384 Free PMC article. Review.
-
Replisome loading reduces chromatin motion independent of DNA synthesis.Elife. 2023 Oct 31;12:RP87572. doi: 10.7554/eLife.87572. Elife. 2023. PMID: 37906089 Free PMC article.
-
Phase-separated super-enhancers confer an innate radioresistance on genomic DNA.J Radiat Res. 2024 Jul 22;65(4):482-490. doi: 10.1093/jrr/rrae044. J Radiat Res. 2024. PMID: 38874522 Free PMC article.
References
Publication types
Grants and funding
LinkOut - more resources
Full Text Sources