High-resolution structures of a thermophilic eukaryotic 80S ribosome reveal atomistic details of translocation
- PMID: 35079002
- PMCID: PMC8789840
- DOI: 10.1038/s41467-022-27967-9
High-resolution structures of a thermophilic eukaryotic 80S ribosome reveal atomistic details of translocation
Abstract
Ribosomes are complex and highly conserved ribonucleoprotein assemblies catalyzing protein biosynthesis in every organism. Here we present high-resolution cryo-EM structures of the 80S ribosome from a thermophilic fungus in two rotational states, which due to increased 80S stability provide a number of mechanistic details of eukaryotic translation. We identify a universally conserved 'nested base-triple knot' in the 26S rRNA at the polypeptide tunnel exit with a bulged-out nucleotide that likely serves as an adaptable element for nascent chain containment and handover. We visualize the structure and dynamics of the ribosome protective factor Stm1 upon ribosomal 40S head swiveling. We describe the structural impact of a unique and essential m1acp3 Ψ 18S rRNA hyper-modification embracing the anticodon wobble-position for eukaryotic tRNA and mRNA translocation. We complete the eEF2-GTPase switch cycle describing the GDP-bound post-hydrolysis state. Taken together, our data and their integration into the structural landscape of 80S ribosomes furthers our understanding of protein biogenesis.
© 2022. The Author(s).
Conflict of interest statement
The authors declare no competing interests.
Figures
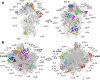
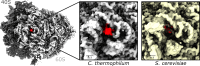
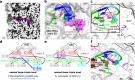
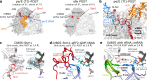
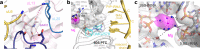
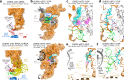
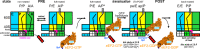
Similar articles
-
Structures of modified eEF2 80S ribosome complexes reveal the role of GTP hydrolysis in translocation.EMBO J. 2007 May 2;26(9):2421-31. doi: 10.1038/sj.emboj.7601677. Epub 2007 Apr 19. EMBO J. 2007. PMID: 17446867 Free PMC article.
-
Domain movements of elongation factor eEF2 and the eukaryotic 80S ribosome facilitate tRNA translocation.EMBO J. 2004 Mar 10;23(5):1008-19. doi: 10.1038/sj.emboj.7600102. Epub 2004 Feb 19. EMBO J. 2004. PMID: 14976550 Free PMC article.
-
Structural Insights into the Role of Diphthamide on Elongation Factor 2 in mRNA Reading-Frame Maintenance.J Mol Biol. 2018 Aug 17;430(17):2677-2687. doi: 10.1016/j.jmb.2018.06.006. Epub 2018 Jun 7. J Mol Biol. 2018. PMID: 29886014
-
Three-dimensional electron cryomicroscopy of ribosomes.Curr Protein Pept Sci. 2002 Feb;3(1):79-91. doi: 10.2174/1389203023380873. Curr Protein Pept Sci. 2002. PMID: 12370013 Review.
-
Atomic structures of the eukaryotic ribosome.Trends Biochem Sci. 2012 May;37(5):189-98. doi: 10.1016/j.tibs.2012.02.007. Epub 2012 Mar 20. Trends Biochem Sci. 2012. PMID: 22436288 Review.
Cited by
-
Non-canonical binding of the Chaetomium thermophilum PolD4 N-terminal PIP motif to PCNA involves Q-pocket and compact 2-fork plug interactions but no 310 helix.FEBS J. 2023 Jan;290(1):162-175. doi: 10.1111/febs.16590. Epub 2022 Aug 22. FEBS J. 2023. PMID: 35942639 Free PMC article.
-
A Short Tale of the Origin of Proteins and Ribosome Evolution.Microorganisms. 2022 Oct 26;10(11):2115. doi: 10.3390/microorganisms10112115. Microorganisms. 2022. PMID: 36363706 Free PMC article. Review.
-
Extended N-Terminal Acetyltransferase Naa50 in Filamentous Fungi Adds to Naa50 Diversity.Int J Mol Sci. 2022 Sep 16;23(18):10805. doi: 10.3390/ijms231810805. Int J Mol Sci. 2022. PMID: 36142717 Free PMC article.
-
Human tumor suppressor protein Pdcd4 binds at the mRNA entry channel in the 40S small ribosomal subunit.Nat Commun. 2024 Aug 8;15(1):6633. doi: 10.1038/s41467-024-50672-8. Nat Commun. 2024. PMID: 39117603 Free PMC article.
-
Engineering tRNAs for the Ribosomal Translation of Non-proteinogenic Monomers.Chem Rev. 2024 May 22;124(10):6444-6500. doi: 10.1021/acs.chemrev.3c00894. Epub 2024 Apr 30. Chem Rev. 2024. PMID: 38688034 Review.
References
-
- Ben-Shem A, et al. The structure of the eukaryotic ribosome at 3.0 A resolution. Science. 2011;334:1524–1529. - PubMed
-
- Anger AM, et al. Structures of the human and Drosophila 80S ribosome. Nature. 2013;497:80–85. - PubMed
-
- Khatter H, Myasnikov AG, Natchiar SK, Klaholz BP. Structure of the human 80S ribosome. Nature. 2015;520:640–645. - PubMed
Publication types
MeSH terms
Substances
Supplementary concepts
LinkOut - more resources
Full Text Sources
Miscellaneous