Advances in Single-Cell Printing
- PMID: 35056245
- PMCID: PMC8778191
- DOI: 10.3390/mi13010080
Advances in Single-Cell Printing
Abstract
Single-cell analysis is becoming an indispensable tool in modern biological and medical research. Single-cell isolation is the key step for single-cell analysis. Single-cell printing shows several distinct advantages among the single-cell isolation techniques, such as precise deposition, high encapsulation efficiency, and easy recovery. Therefore, recent developments in single-cell printing have attracted extensive attention. We review herein the recently developed bioprinting strategies with single-cell resolution, with a special focus on inkjet-like single-cell printing. First, we discuss the common cell printing strategies and introduce several typical and advanced printing strategies. Then, we introduce several typical applications based on single-cell printing, from single-cell array screening and mass spectrometry-based single-cell analysis to three-dimensional tissue formation. In the last part, we discuss the pros and cons of the single-cell strategies and provide a brief outlook for single-cell printing.
Keywords: cell array; inkjet printing; microfluidics; screening; single-cell analysis.
Conflict of interest statement
The authors declare no conflict of interest.
Figures
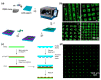
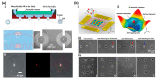
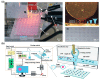
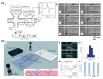
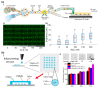
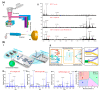
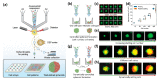
Similar articles
-
Inkjet Bioprinting of Biomaterials.Chem Rev. 2020 Oct 14;120(19):10793-10833. doi: 10.1021/acs.chemrev.0c00008. Epub 2020 Sep 9. Chem Rev. 2020. PMID: 32902959 Review.
-
Advances in tissue engineering of vasculature through three-dimensional bioprinting.Dev Dyn. 2021 Dec;250(12):1717-1738. doi: 10.1002/dvdy.385. Epub 2021 Jul 2. Dev Dyn. 2021. PMID: 34115420 Review.
-
Inkjet Printing and 3D Printing Strategies for Biosensing, Analytical, and Diagnostic Applications.Adv Mater. 2022 Aug;34(31):e2105015. doi: 10.1002/adma.202105015. Epub 2022 Jun 17. Adv Mater. 2022. PMID: 35338719 Review.
-
Three-dimensional bioprinting in tissue engineering and regenerative medicine.Biotechnol Lett. 2016 Feb;38(2):203-11. doi: 10.1007/s10529-015-1975-1. Epub 2015 Oct 14. Biotechnol Lett. 2016. PMID: 26466597 Review.
-
Inkjet-Printing Patterned Chip on Sticky Superhydrophobic Surface for High-Efficiency Single-Cell Array Trapping and Real-Time Observation of Cellular Apoptosis.ACS Appl Mater Interfaces. 2018 Sep 19;10(37):31054-31060. doi: 10.1021/acsami.8b10703. Epub 2018 Sep 10. ACS Appl Mater Interfaces. 2018. PMID: 30148358
Cited by
-
Laser microdissection system based on structured light modulation dual cutting mode and negative pressure adsorption collection.PLoS One. 2024 Aug 26;19(8):e0308662. doi: 10.1371/journal.pone.0308662. eCollection 2024. PLoS One. 2024. PMID: 39186704 Free PMC article.
-
Single-Cell Microarray Chip with Inverse-Tapered Wells to Maintain High Ratio of Cell Trapping.Micromachines (Basel). 2023 Feb 20;14(2):492. doi: 10.3390/mi14020492. Micromachines (Basel). 2023. PMID: 36838192 Free PMC article.
-
The 3Rs in Experimental Liver Disease.Animals (Basel). 2023 Jul 19;13(14):2357. doi: 10.3390/ani13142357. Animals (Basel). 2023. PMID: 37508134 Free PMC article. Review.
-
Investigation of piezoelectric printing devices for oil-free and on-demand picolitre monodisperse droplet generation.Sci Rep. 2024 Jul 23;14(1):17104. doi: 10.1038/s41598-024-67849-2. Sci Rep. 2024. PMID: 39048610 Free PMC article.
-
The Effect of Ink Supply Pressure on Piezoelectric Inkjet.Micromachines (Basel). 2022 Apr 14;13(4):615. doi: 10.3390/mi13040615. Micromachines (Basel). 2022. PMID: 35457919 Free PMC article.
References
Publication types
Grants and funding
LinkOut - more resources
Full Text Sources
Other Literature Sources
Miscellaneous