An ESCRT/VPS4 Envelopment Trap To Examine the Mechanism of Alphaherpesvirus Assembly and Transport in Neurons
- PMID: 35045266
- PMCID: PMC8941884
- DOI: 10.1128/jvi.02178-21
An ESCRT/VPS4 Envelopment Trap To Examine the Mechanism of Alphaherpesvirus Assembly and Transport in Neurons
Abstract
The assembly and egress of alphaherpesviruses, including herpes simplex virus 1 (HSV-1) and pseudorabies virus (PRV), within neurons is poorly understood. A key unresolved question is the structure of the viral particle that moves by anterograde transport along the axon, and two alternative mechanisms have been described. In the "married" model, capsids acquire their envelopes in the cell body and then traffic along axons as enveloped virions within a bounding organelle. In the "separate" model, nonenveloped capsids travel from the cell body into and along the axon, eventually encountering their envelopment organelles at a distal site, such as the nerve cell terminal. Here, we describe an "envelopment trap" to test these models using the dominant negative terminal endosomal sorting complex required for transport (ESCRT) component VPS4-EQ. Green fluorescent protein (GFP)-tagged VPS4-EQ was used to arrest HSV-1 or PRV capsid envelopment, inhibit downstream trafficking, and GFP-label envelopment intermediates. We found that GFP-VPS4-EQ inhibited trafficking of HSV-1 capsids into and along the neurites and axons of mouse CAD cells and rat embryonic primary cortical neurons, consistent with egress via the married pathway. In contrast, transport of HSV-1 capsids was unaffected in the neurites of human SK-N-SH neuroblastoma cells, consistent with the separate mechanism. Unexpectedly, PRV (generally thought to utilize the married pathway) also appeared to employ the separate mechanism in SK-N-SH cells. We propose that apparent differences in the methods of HSV-1 and PRV egress are more likely a reflection of the host neuron in which transport is studied rather than true biological differences between the viruses themselves. IMPORTANCE Alphaherpesviruses, including herpes simplex virus 1 (HSV-1) and pseudorabies virus (PRV), are pathogens of the nervous system. They replicate in the nerve cell body and then travel great distances along axons to reach nerve termini and spread to adjacent epithelial cells; however, key aspects of how these viruses travel along axons remain controversial. Here, we test two alternative mechanisms for transport, the married and separate models, by blocking envelope assembly, a critical step in viral egress. When we arrest formation of the viral envelope using a mutated component of the cellular ESCRT apparatus, we find that entry of viral particles into axons is blocked in some types of neurons but not others. This approach allows us to determine whether envelope assembly occurs prior to entry of viruses into axons or afterwards and, thus, to distinguish between the alternative models for viral transport.
Keywords: ESCRT; HSV-1; PRV; anterograde transport; envelopment; neuronal egress.
Conflict of interest statement
The authors declare no conflict of interest.
Figures
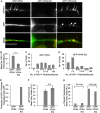
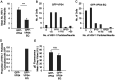
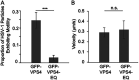
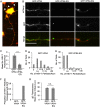
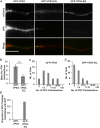
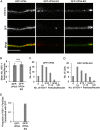
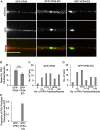
Similar articles
-
Blocking ESCRT-mediated envelopment inhibits microtubule-dependent trafficking of alphaherpesviruses in vitro.J Virol. 2014 Dec;88(24):14467-78. doi: 10.1128/JVI.02777-14. Epub 2014 Oct 8. J Virol. 2014. PMID: 25297998 Free PMC article.
-
Herpes Simplex Virus Capsid Localization to ESCRT-VPS4 Complexes in the Presence and Absence of the Large Tegument Protein UL36p.J Virol. 2016 Jul 27;90(16):7257-7267. doi: 10.1128/JVI.00857-16. Print 2016 Aug 15. J Virol. 2016. PMID: 27252536 Free PMC article.
-
Completely assembled virus particles detected by transmission electron microscopy in proximal and mid-axons of neurons infected with herpes simplex virus type 1, herpes simplex virus type 2 and pseudorabies virus.Virology. 2011 Jan 5;409(1):12-6. doi: 10.1016/j.virol.2010.10.009. Epub 2010 Oct 30. Virology. 2011. PMID: 21036381 Free PMC article.
-
Transport and egress of herpes simplex virus in neurons.Rev Med Virol. 2008 Jan-Feb;18(1):35-51. doi: 10.1002/rmv.560. Rev Med Virol. 2008. PMID: 17992661 Review.
-
HSV-1 Cytoplasmic Envelopment and Egress.Int J Mol Sci. 2020 Aug 19;21(17):5969. doi: 10.3390/ijms21175969. Int J Mol Sci. 2020. PMID: 32825127 Free PMC article. Review.
References
Publication types
MeSH terms
Substances
Grants and funding
LinkOut - more resources
Full Text Sources
Miscellaneous