Telomerase Reverse Transcriptase Preserves Neuron Survival and Cognition in Alzheimer's Disease Models
- PMID: 35036927
- PMCID: PMC8759755
- DOI: 10.1038/s43587-021-00146-z
Telomerase Reverse Transcriptase Preserves Neuron Survival and Cognition in Alzheimer's Disease Models
Abstract
Amyloid-induced neurodegeneration plays a central role in Alzheimer's disease (AD) pathogenesis. Here, we show that telomerase reverse transcriptase (TERT) haploinsufficiency decreases BDNF and increases amyloid-β (Aβ) precursor in murine brain. Moreover, prior to disease onset, the TERT locus sustains accumulation of repressive epigenetic marks in murine and human AD neurons, implicating TERT repression in amyloid-induced neurodegeneration. To test the impact of sustained TERT expression on AD pathobiology, AD mouse models were engineered to maintain physiological levels of TERT in adult neurons, resulting in reduced Aβ accumulation, improved spine morphology, and preserved cognitive function. Mechanistically, integrated profiling revealed that TERT interacts with β-catenin and RNA polymerase II at gene promoters and upregulates gene networks governing synaptic signaling and learning processes. These TERT-directed transcriptional activities do not require its catalytic activity nor telomerase RNA. These findings provide genetic proof-of-concept for somatic TERT gene activation therapy in attenuating AD progression including cognitive decline.
Figures
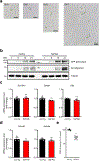
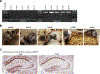
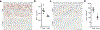
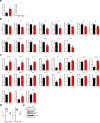
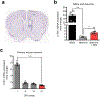

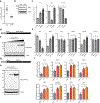
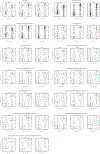
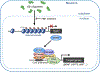
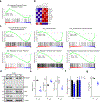
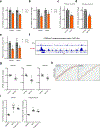
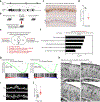
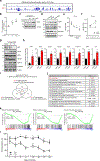
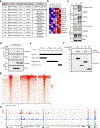
Comment in
-
Counteracting Alzheimer's disease via somatic TERT activation.Nat Aging. 2021 Dec;1(12):1081-1082. doi: 10.1038/s43587-021-00145-0. Nat Aging. 2021. PMID: 37117526 No abstract available.
Similar articles
-
The catalytic subunit of telomerase protects neurons against amyloid beta-peptide-induced apoptosis.J Neurochem. 2000 Jul;75(1):117-24. doi: 10.1046/j.1471-4159.2000.0750117.x. J Neurochem. 2000. PMID: 10854254
-
Neuronal Protein Tyrosine Phosphatase 1B Hastens Amyloid β-Associated Alzheimer's Disease in Mice.J Neurosci. 2020 Feb 12;40(7):1581-1593. doi: 10.1523/JNEUROSCI.2120-19.2019. Epub 2020 Jan 8. J Neurosci. 2020. PMID: 31915254 Free PMC article.
-
Blocking IGF Signaling in Adult Neurons Alleviates Alzheimer's Disease Pathology through Amyloid-β Clearance.J Neurosci. 2015 Aug 19;35(33):11500-13. doi: 10.1523/JNEUROSCI.0343-15.2015. J Neurosci. 2015. PMID: 26290229 Free PMC article.
-
Low density lipoprotein receptor-related proteins (LRPs), Alzheimer's and cognition.Curr Drug Targets CNS Neurol Disord. 2005 Oct;4(5):469-80. doi: 10.2174/156800705774322102. Curr Drug Targets CNS Neurol Disord. 2005. PMID: 16266282 Review.
-
Parenchymal and vascular Abeta-deposition and its effects on the degeneration of neurons and cognition in Alzheimer's disease.J Cell Mol Med. 2008 Oct;12(5B):1848-62. doi: 10.1111/j.1582-4934.2008.00411.x. Epub 2008 Jul 10. J Cell Mol Med. 2008. PMID: 18624777 Free PMC article. Review.
Cited by
-
Exploring the Causal Relationship Between Telomere Biology and Alzheimer's Disease.Mol Neurobiol. 2023 Aug;60(8):4169-4183. doi: 10.1007/s12035-023-03337-4. Epub 2023 Apr 12. Mol Neurobiol. 2023. PMID: 37046137 Free PMC article. Review.
-
Gene expression in mice with endothelium-specific telomerase knockout.Front Cell Dev Biol. 2023 Dec 7;11:1295072. doi: 10.3389/fcell.2023.1295072. eCollection 2023. Front Cell Dev Biol. 2023. PMID: 38161328 Free PMC article. No abstract available.
-
The STIM1/2-Regulated Calcium Homeostasis Is Impaired in Hippocampal Neurons of the 5xFAD Mouse Model of Alzheimer's Disease.Int J Mol Sci. 2022 Nov 26;23(23):14810. doi: 10.3390/ijms232314810. Int J Mol Sci. 2022. PMID: 36499137 Free PMC article.
-
Ageing in the brain: mechanisms and rejuvenating strategies.Cell Mol Life Sci. 2023 Jun 24;80(7):190. doi: 10.1007/s00018-023-04832-6. Cell Mol Life Sci. 2023. PMID: 37354261 Free PMC article. Review.
-
Chemobrain: An accelerated aging process linking adenosine A2A receptor signaling in cancer survivors.Int Rev Neurobiol. 2023;170:267-305. doi: 10.1016/bs.irn.2023.08.003. Epub 2023 Aug 25. Int Rev Neurobiol. 2023. PMID: 37741694 Free PMC article. Review.
References
Publication types
MeSH terms
Substances
Grants and funding
LinkOut - more resources
Full Text Sources
Medical
Molecular Biology Databases