Transposable element activation promotes neurodegeneration in a Drosophila model of Huntington's disease
- PMID: 35036881
- PMCID: PMC8752904
- DOI: 10.1016/j.isci.2021.103702
Transposable element activation promotes neurodegeneration in a Drosophila model of Huntington's disease
Abstract
Huntington's disease (HD) is an autosomal dominant disorder with progressive motor dysfunction and cognitive decline. The disease is caused by a CAG repeat expansion in the IT15 gene, which elongates a polyglutamine stretch of the HD protein, Huntingtin. No therapeutic treatments are available, and new pharmacological targets are needed. Retrotransposons are transposable elements (TEs) that represent 40% and 30% of the human and Drosophila genomes and replicate through an RNA intermediate. Mounting evidence suggests that mammalian TEs are active during neurogenesis and may be involved in diseases of the nervous system. Here we show that TE expression and mobilization are increased in a Drosophila melanogaster HD model. By inhibiting TE mobilization with Reverse Transcriptase inhibitors, polyQ-dependent eye neurodegeneration and genome instability in larval brains are rescued and fly lifespan is increased. These results suggest that TE activation may be involved in polyQ-induced neurotoxicity and a potential pharmacological target.
Keywords: Biological sciences; Molecular biology; Neuroscience.
© 2021 The Author(s).
Conflict of interest statement
The authors declare no competing interests.
Figures
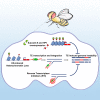
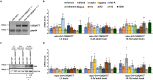
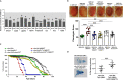
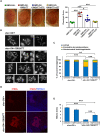
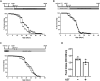
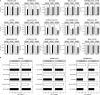
Similar articles
-
Analysis of LINE1 Retrotransposons in Huntington's Disease.Front Cell Neurosci. 2022 Jan 14;15:743797. doi: 10.3389/fncel.2021.743797. eCollection 2021. Front Cell Neurosci. 2022. PMID: 35095420 Free PMC article.
-
Amelioration of Huntington's disease phenotype in astrocytes derived from iPSC-derived neural progenitor cells of Huntington's disease monkeys.PLoS One. 2019 Mar 21;14(3):e0214156. doi: 10.1371/journal.pone.0214156. eCollection 2019. PLoS One. 2019. PMID: 30897183 Free PMC article.
-
Striatal Vulnerability in Huntington's Disease: Neuroprotection Versus Neurotoxicity.Brain Sci. 2017 Jun 7;7(6):63. doi: 10.3390/brainsci7060063. Brain Sci. 2017. PMID: 28590448 Free PMC article. Review.
-
Methylene blue modulates huntingtin aggregation intermediates and is protective in Huntington's disease models.J Neurosci. 2012 Aug 8;32(32):11109-19. doi: 10.1523/JNEUROSCI.0895-12.2012. J Neurosci. 2012. PMID: 22875942 Free PMC article.
-
Insights into White Matter Defect in Huntington's Disease.Cells. 2022 Oct 26;11(21):3381. doi: 10.3390/cells11213381. Cells. 2022. PMID: 36359783 Free PMC article. Review.
Cited by
-
Expression of Transposable Elements in the Brain of the Drosophila melanogaster Model for Fragile X Syndrome.Genes (Basel). 2023 May 9;14(5):1060. doi: 10.3390/genes14051060. Genes (Basel). 2023. PMID: 37239420 Free PMC article.
-
Drosophila as a Model for Human Viral Neuroinfections.Cells. 2022 Aug 29;11(17):2685. doi: 10.3390/cells11172685. Cells. 2022. PMID: 36078091 Free PMC article. Review.
-
Dysregulated Expression of Transposable Elements in TDP-43M337V Human Motor Neurons That Recapitulate Amyotrophic Lateral Sclerosis In Vitro.Int J Mol Sci. 2022 Dec 19;23(24):16222. doi: 10.3390/ijms232416222. Int J Mol Sci. 2022. PMID: 36555863 Free PMC article.
-
Neuroprotective Effects of PARP Inhibitors in Drosophila Models of Alzheimer's Disease.Cells. 2022 Apr 9;11(8):1284. doi: 10.3390/cells11081284. Cells. 2022. PMID: 35455964 Free PMC article.
-
Cytological heterogeneity of heterochromatin among 10 sequenced Drosophila species.Genetics. 2022 Sep 30;222(2):iyac119. doi: 10.1093/genetics/iyac119. Genetics. 2022. PMID: 35946576 Free PMC article.
References
-
- Andrews S. Babraham Bioinformatic; 2010. FASTQC. A Quality Control Tool for High Throughput Sequence Data. Available online at: http://www.bioinformatics.babraham.ac.uk/projects/fastqc/
LinkOut - more resources
Full Text Sources
Molecular Biology Databases