The Role of Even-Skipped in Drosophila Larval Somatosensory Circuit Assembly
- PMID: 35031555
- PMCID: PMC8856706
- DOI: 10.1523/ENEURO.0403-21.2021
The Role of Even-Skipped in Drosophila Larval Somatosensory Circuit Assembly
Abstract
Proper somatosensory circuit assembly is critical for processing somatosensory stimuli and for responding accordingly. In comparison to other sensory circuits (e.g., olfactory and visual), somatosensory circuits have unique anatomy and function. However, understanding of somatosensory circuit development lags far behind that of other sensory systems. For example, there are few identified transcription factors required for integration of interneurons into functional somatosensory circuits. Here, as a model, we examine one type of somatosensory interneuron, Even-skipped (Eve) expressing laterally placed interneurons (ELs) of the Drosophila larval nerve cord. Eve is a highly conserved, homeodomain transcription factor known to play a role in cell fate specification and neuronal axon guidance. Because marker genes are often functionally important in the cell types they define, we deleted eve expression specifically from EL interneurons. On the cell biological level, using single neuron labeling, we find eve plays several previously undescribed roles in refinement of neuron morphogenesis. Eve suppresses aberrant neurite branching, promotes axon elongation, and regulates dorsal-ventral dendrite position. On the circuit level, using optogenetics, calcium imaging, and behavioral analysis, we find eve expression is required in EL interneurons for the normal encoding of somatosensory stimuli and for normal mapping of outputs to behavior. We conclude that the eve gene product coordinately regulates multiple aspects of EL interneuron morphogenesis and is critically required to properly integrate EL interneurons into somatosensory circuits. Our data shed light on the genetic regulation of somatosensory circuit assembly.
Keywords: embryo; larva; mechanosensation; proprioception; somatosensation.
Copyright © 2022 Marshall and Heckscher.
Figures
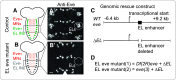
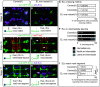
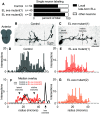
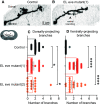
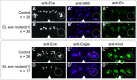
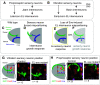
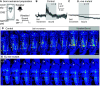
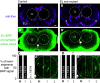
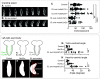
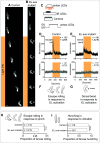
Similar articles
-
Even-Skipped(+) Interneurons Are Core Components of a Sensorimotor Circuit that Maintains Left-Right Symmetric Muscle Contraction Amplitude.Neuron. 2015 Oct 21;88(2):314-29. doi: 10.1016/j.neuron.2015.09.009. Epub 2015 Oct 1. Neuron. 2015. PMID: 26439528 Free PMC article.
-
dbx mediates neuronal specification and differentiation through cross-repressive, lineage-specific interactions with eve and hb9.Development. 2009 Oct;136(19):3257-66. doi: 10.1242/dev.037242. Epub 2009 Aug 26. Development. 2009. PMID: 19710170 Free PMC article.
-
The homeobox transcription factor Even-skipped regulates acquisition of electrical properties in Drosophila neurons.Neural Dev. 2006 Nov 16;1:3. doi: 10.1186/1749-8104-1-3. Neural Dev. 2006. PMID: 17147779 Free PMC article.
-
Transparent Touch: Insights From Model Systems on Epidermal Control of Somatosensory Innervation.Front Cell Neurosci. 2021 May 31;15:680345. doi: 10.3389/fncel.2021.680345. eCollection 2021. Front Cell Neurosci. 2021. PMID: 34135734 Free PMC article. Review.
-
The two-body problem: Proprioception and motor control across the metamorphic divide.Curr Opin Neurobiol. 2022 Jun;74:102546. doi: 10.1016/j.conb.2022.102546. Epub 2022 May 2. Curr Opin Neurobiol. 2022. PMID: 35512562 Free PMC article. Review.
Cited by
-
CRASH2p: Closed-loop Two Photon Imaging in a Freely Moving Animal.bioRxiv [Preprint]. 2024 Dec 13:2024.05.22.595209. doi: 10.1101/2024.05.22.595209. bioRxiv. 2024. PMID: 38826435 Free PMC article. Preprint.
-
scRNA-seq data from the larval Drosophila ventral cord provides a resource for studying motor systems function and development.Dev Cell. 2024 May 6;59(9):1210-1230.e9. doi: 10.1016/j.devcel.2024.03.016. Epub 2024 Apr 2. Dev Cell. 2024. PMID: 38569548
-
Sequential addition of neuronal stem cell temporal cohorts generates a feed-forward circuit in the Drosophila larval nerve cord.Elife. 2022 Jun 20;11:e79276. doi: 10.7554/eLife.79276. Elife. 2022. PMID: 35723253 Free PMC article.
References
Publication types
MeSH terms
Substances
Grants and funding
LinkOut - more resources
Full Text Sources
Molecular Biology Databases