Cryo-Electron Microscopy and Biochemical Analysis Offer Insights Into the Effects of Acidic pH, Such as Occur During Acidosis, on the Complement Binding Properties of C-Reactive Protein
- PMID: 34975846
- PMCID: PMC8716467
- DOI: 10.3389/fimmu.2021.757633
Cryo-Electron Microscopy and Biochemical Analysis Offer Insights Into the Effects of Acidic pH, Such as Occur During Acidosis, on the Complement Binding Properties of C-Reactive Protein
Abstract
The pentraxin family of proteins includes C-reactive protein (CRP), a canonical marker for the acute phase inflammatory response. As compared to normal physiological conditions in human serum, under conditions associated with damage and inflammation, such as acidosis and the oxidative burst, CRP exhibits modulated biochemical properties that may have a structural basis. Here, we explore how pH and ligand binding affect the structure and biochemical properties of CRP. Cryo-electron microscopy was used to solve structures of CRP at pH 7.5 or pH 5 and in the presence or absence of the ligand phosphocholine (PCh), which yielded 7 new high-resolution structures of CRP, including pentameric and decameric complexes. Structures previously derived from crystallography were imperfect pentagons, as shown by the variable angles between each subunit, whereas pentameric CRP derived from cryoEM was found to have C5 symmetry, with subunits forming a regular pentagon with equal angles. This discrepancy indicates flexibility at the interfaces of monomers that may relate to activation of the complement system by the C1 complex. CRP also appears to readily decamerise in solution into dimers of pentamers, which obscures the postulated binding sites for C1. Subtle structural rearrangements were observed between the conditions tested, including a putative change in histidine protonation that may prime the disulphide bridges for reduction and enhanced ability to activate the immune system. Enzyme-linked immunosorbent assays showed that CRP had markedly increased association to the C1 complex and immunoglobulins under conditions associated with acidosis, whilst a reduction in the Ca2+ concentration lowered this pH-sensitivity for C1q, but not immunoglobulins, suggesting different modes of binding. These data suggest a model whereby a change in the ionic nature of CRP and immunological proteins can make it more adhesive to potential ligands without large structural rearrangements.
Keywords: CRP; ELISA; acidosis; complement; cryoEM; structural biology.
Copyright © 2021 Noone, van der Velden and Sharp.
Conflict of interest statement
The authors declare that the research was conducted in the absence of any commercial or financial relationships that could be construed as a potential conflict of interest.
Figures
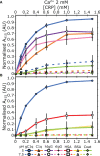

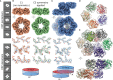
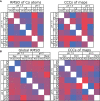
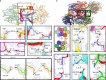
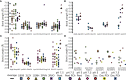
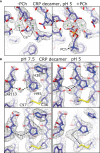
Similar articles
-
Identification of acidic pH-dependent ligands of pentameric C-reactive protein.J Biol Chem. 2010 Nov 12;285(46):36235-44. doi: 10.1074/jbc.M110.142026. Epub 2010 Sep 14. J Biol Chem. 2010. PMID: 20843812 Free PMC article.
-
Structural basis for surface activation of the classical complement cascade by the short pentraxin C-reactive protein.Proc Natl Acad Sci U S A. 2024 Sep 10;121(37):e2404542121. doi: 10.1073/pnas.2404542121. Epub 2024 Sep 6. Proc Natl Acad Sci U S A. 2024. PMID: 39240968
-
Studies on the interactions between C-reactive protein and complement proteins.Immunology. 2007 May;121(1):40-50. doi: 10.1111/j.1365-2567.2007.02535.x. Epub 2007 Jan 18. Immunology. 2007. PMID: 17244159 Free PMC article.
-
C-Reactive Protein and Its Structural Isoforms: An Evolutionary Conserved Marker and Central Player in Inflammatory Diseases and Beyond.Subcell Biochem. 2020;94:499-520. doi: 10.1007/978-3-030-41769-7_20. Subcell Biochem. 2020. PMID: 32189313 Review.
-
Structure-Function Relationships of C-Reactive Protein in Bacterial Infection.Front Immunol. 2019 Feb 26;10:166. doi: 10.3389/fimmu.2019.00166. eCollection 2019. Front Immunol. 2019. PMID: 30863393 Free PMC article. Review.
Cited by
-
PTX3 structure determination using a hybrid cryoelectron microscopy and AlphaFold approach offers insights into ligand binding and complement activation.Proc Natl Acad Sci U S A. 2022 Aug 16;119(33):e2208144119. doi: 10.1073/pnas.2208144119. Epub 2022 Aug 8. Proc Natl Acad Sci U S A. 2022. PMID: 35939690 Free PMC article.
-
An evolutionarily conserved function of C-reactive protein is to prevent the formation of amyloid fibrils.Front Immunol. 2024 Sep 16;15:1466865. doi: 10.3389/fimmu.2024.1466865. eCollection 2024. Front Immunol. 2024. PMID: 39351235 Free PMC article.
-
Editorial: Interactions of Pentraxins and Complement in Infection, Inflammation, and Cancer.Front Immunol. 2022 Feb 17;13:861359. doi: 10.3389/fimmu.2022.861359. eCollection 2022. Front Immunol. 2022. PMID: 35251053 Free PMC article. No abstract available.
-
C-reactive protein: structure, function, regulation, and role in clinical diseases.Front Immunol. 2024 Jun 14;15:1425168. doi: 10.3389/fimmu.2024.1425168. eCollection 2024. Front Immunol. 2024. PMID: 38947332 Free PMC article. Review.
-
Lifting the Concentration Limit of Mass Photometry by PEG Nanopatterning.Nano Lett. 2024 Aug 21;24(33):10032-10039. doi: 10.1021/acs.nanolett.4c01667. Epub 2024 Jul 1. Nano Lett. 2024. PMID: 38950386 Free PMC article.
References
Publication types
MeSH terms
Substances
LinkOut - more resources
Full Text Sources
Research Materials
Miscellaneous