S-adenosylmethionine-dependent methyltransferase inhibitor DZNep blocks transcription and translation of SARS-CoV-2 genome with a low tendency to select for drug-resistant viral variants
- PMID: 34968527
- PMCID: PMC8714615
- DOI: 10.1016/j.antiviral.2021.105232
S-adenosylmethionine-dependent methyltransferase inhibitor DZNep blocks transcription and translation of SARS-CoV-2 genome with a low tendency to select for drug-resistant viral variants
Abstract
We report the in vitro antiviral activity of DZNep (3-Deazaneplanocin A; an inhibitor of S-adenosylmethionine-dependent methyltransferase) against SARS-CoV-2, besides demonstrating its protective efficacy against lethal infection of infectious bronchitis virus (IBV, a member of the Coronaviridae family). DZNep treatment resulted in reduced synthesis of SARS-CoV-2 RNA and proteins without affecting other steps of viral life cycle. We demonstrated that deposition of N6-methyl adenosine (m6A) in SARS-CoV-2 RNA in the infected cells recruits heterogeneous nuclear ribonucleoprotein A1 (hnRNPA1), an RNA binding protein which serves as a m6A reader. DZNep inhibited the recruitment of hnRNPA1 at m6A-modified SARS-CoV-2 RNA which eventually suppressed the synthesis of the viral genome. In addition, m6A-marked RNA and hnRNPA1 interaction was also shown to regulate early translation to replication switch of SARS-CoV-2 genome. Furthermore, abrogation of methylation by DZNep also resulted in defective synthesis of the 5' cap of viral RNA, thereby resulting in its failure to interact with eIF4E (a cap-binding protein), eventually leading to a decreased synthesis of viral proteins. Most importantly, DZNep-resistant mutants could not be observed upon long-term sequential passage of SARS-CoV-2 in cell culture. In summary, we report the novel role of methylation in the life cycle of SARS-CoV-2 and propose that targeting the methylome using DZNep could be of significant therapeutic value against SARS-CoV-2 infection.
Keywords: DZNep; Drug resistance; Epitranscriptomic; SARS-CoV-2; Virus replication.
Copyright © 2021 Elsevier B.V. All rights reserved.
Conflict of interest statement
The authors declare that they have no known competing financial interests or personal relationships that could have appeared to influence the work reported in this paper.
Figures
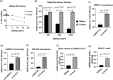
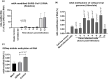
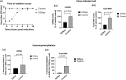
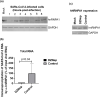
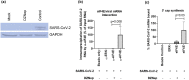
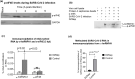
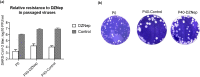
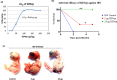
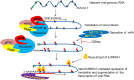
Similar articles
-
Emetine suppresses SARS-CoV-2 replication by inhibiting interaction of viral mRNA with eIF4E.Antiviral Res. 2021 May;189:105056. doi: 10.1016/j.antiviral.2021.105056. Epub 2021 Mar 10. Antiviral Res. 2021. PMID: 33711336 Free PMC article.
-
Mechanisms of anti-vesicular stomatitis virus activity of deazaneplanocin and its 3-brominated analogs.Antiviral Res. 2021 Jul;191:105088. doi: 10.1016/j.antiviral.2021.105088. Epub 2021 May 19. Antiviral Res. 2021. PMID: 34019950 Free PMC article.
-
Direct RNA Sequencing Reveals SARS-CoV-2 m6A Sites and Possible Differential DRACH Motif Methylation among Variants.Viruses. 2021 Oct 20;13(11):2108. doi: 10.3390/v13112108. Viruses. 2021. PMID: 34834915 Free PMC article.
-
3-Deazaneplanocin A (DZNep): A Drug That Deserves a Second Look.J Med Chem. 2024 Oct 24;67(20):17964-17979. doi: 10.1021/acs.jmedchem.4c01566. Epub 2024 Oct 11. J Med Chem. 2024. PMID: 39392180 Review.
-
The multifarious roles of heterogeneous ribonucleoprotein A1 in viral infections.Rev Med Virol. 2020 Mar;30(2):e2097. doi: 10.1002/rmv.2097. Epub 2020 Jan 27. Rev Med Virol. 2020. PMID: 31989716 Free PMC article. Review.
Cited by
-
Multiple functions of heterogeneous nuclear ribonucleoproteins in the positive single-stranded RNA virus life cycle.Front Immunol. 2022 Sep 2;13:989298. doi: 10.3389/fimmu.2022.989298. eCollection 2022. Front Immunol. 2022. PMID: 36119073 Free PMC article. Review.
-
High-sensitivity profiling of SARS-CoV-2 noncoding region-host protein interactome reveals the potential regulatory role of negative-sense viral RNA.mSystems. 2023 Aug 31;8(4):e0013523. doi: 10.1128/msystems.00135-23. Epub 2023 Jun 14. mSystems. 2023. PMID: 37314180 Free PMC article.
-
Sulfoglycodendron Antivirals with Scalable Architectures and Activities.bioRxiv [Preprint]. 2024 Aug 18:2024.08.01.606251. doi: 10.1101/2024.08.01.606251. bioRxiv. 2024. Update in: J Chem Inf Model. 2024 Sep 23;64(18):7141-7151. doi: 10.1021/acs.jcim.4c00541. PMID: 39131386 Free PMC article. Updated. Preprint.
-
The risk of COVID-19 can be predicted by a nomogram based on m6A-related genes.Infect Genet Evol. 2022 Dec;106:105389. doi: 10.1016/j.meegid.2022.105389. Epub 2022 Nov 29. Infect Genet Evol. 2022. PMID: 36460278 Free PMC article.
-
Melatonin: Regulation of Viral Phase Separation and Epitranscriptomics in Post-Acute Sequelae of COVID-19.Int J Mol Sci. 2022 Jul 23;23(15):8122. doi: 10.3390/ijms23158122. Int J Mol Sci. 2022. PMID: 35897696 Free PMC article. Review.
References
-
- Boccaletto P., Baginski B. MODOMICS: an operational guide to the use of the RNA modification pathways database. Methods Mol. Biol. 2021;2284:481–505. - PubMed
-
- Brocard M., Ruggieri A., Locker N. m6A RNA methylation, a new hallmark in virus-host interactions. J. Gen. Virol. 2017;98:2207–2214. - PubMed
-
- Burgess H.M., Depledge D.P., Thompson L., Srinivas K.P., Grande R.C., Vink E.I., Abebe J.S., Blackaby W.P., Hendrick A., Albertella M.R., Kouzarides T., Stapleford K.A., Wilson A.C., Mohr I. Targeting the m(6)A RNA modification pathway blocks SARS-CoV-2 and HCoV-OC43 replication. Genes Dev. 2021;35:1005–1019. - PMC - PubMed
Publication types
MeSH terms
Substances
LinkOut - more resources
Full Text Sources
Miscellaneous