CryoEM of RUVBL1-RUVBL2-ZNHIT2, a complex that interacts with pre-mRNA-processing-splicing factor 8
- PMID: 34951455
- PMCID: PMC8789047
- DOI: 10.1093/nar/gkab1267
CryoEM of RUVBL1-RUVBL2-ZNHIT2, a complex that interacts with pre-mRNA-processing-splicing factor 8
Abstract
Biogenesis of the U5 small nuclear ribonucleoprotein (snRNP) is an essential and highly regulated process. In particular, PRPF8, one of U5 snRNP main components, requires HSP90 working in concert with R2TP, a cochaperone complex containing RUVBL1 and RUVBL2 AAA-ATPases, and additional factors that are still poorly characterized. Here, we use biochemistry, interaction mapping, mass spectrometry and cryoEM to study the role of ZNHIT2 in the regulation of the R2TP chaperone during the biogenesis of PRPF8. ZNHIT2 forms a complex with R2TP which depends exclusively on the direct interaction of ZNHIT2 with the RUVBL1-RUVBL2 ATPases. The cryoEM analysis of this complex reveals that ZNHIT2 alters the conformation and nucleotide state of RUVBL1-RUVBL2, affecting its ATPase activity. We characterized the interactions between R2TP, PRPF8, ZNHIT2, ECD and AAR2 proteins. Interestingly, PRPF8 makes a direct interaction with R2TP and this complex can incorporate ZNHIT2 and other proteins involved in the biogenesis of PRPF8 such as ECD and AAR2. Together, these results show that ZNHIT2 participates in the assembly of the U5 snRNP as part of a network of contacts between assembly factors required for PRPF8 biogenesis and the R2TP-HSP90 chaperone, while concomitantly regulating the structure and nucleotide state of R2TP.
© The Author(s) 2021. Published by Oxford University Press on behalf of Nucleic Acids Research.
Figures
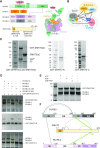
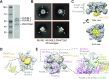

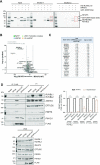
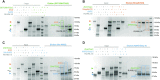
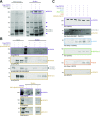
Similar articles
-
R2TP/Prefoldin-like component RUVBL1/RUVBL2 directly interacts with ZNHIT2 to regulate assembly of U5 small nuclear ribonucleoprotein.Nat Commun. 2017 May 31;8:15615. doi: 10.1038/ncomms15615. Nat Commun. 2017. PMID: 28561026 Free PMC article.
-
Assembly of the U5 snRNP component PRPF8 is controlled by the HSP90/R2TP chaperones.J Cell Biol. 2017 Jun 5;216(6):1579-1596. doi: 10.1083/jcb.201701165. Epub 2017 May 17. J Cell Biol. 2017. PMID: 28515276 Free PMC article.
-
Structural mechanism for regulation of the AAA-ATPases RUVBL1-RUVBL2 in the R2TP co-chaperone revealed by cryo-EM.Sci Adv. 2019 May 1;5(5):eaaw1616. doi: 10.1126/sciadv.aaw1616. eCollection 2019 May. Sci Adv. 2019. PMID: 31049401 Free PMC article.
-
RUVBL1-RUVBL2 AAA-ATPase: a versatile scaffold for multiple complexes and functions.Curr Opin Struct Biol. 2021 Apr;67:78-85. doi: 10.1016/j.sbi.2020.08.010. Epub 2020 Oct 28. Curr Opin Struct Biol. 2021. PMID: 33129013 Review.
-
RPAP3 C-Terminal Domain: A Conserved Domain for the Assembly of R2TP Co-Chaperone Complexes.Cells. 2020 May 6;9(5):1139. doi: 10.3390/cells9051139. Cells. 2020. PMID: 32384603 Free PMC article. Review.
Cited by
-
Structure of the human 20S U5 snRNP.Nat Struct Mol Biol. 2024 May;31(5):752-756. doi: 10.1038/s41594-024-01250-5. Epub 2024 Mar 11. Nat Struct Mol Biol. 2024. PMID: 38467877 Free PMC article.
-
The Role of Hsp90-R2TP in Macromolecular Complex Assembly and Stabilization.Biomolecules. 2022 Jul 28;12(8):1045. doi: 10.3390/biom12081045. Biomolecules. 2022. PMID: 36008939 Free PMC article. Review.
-
Roodmus: a toolkit for benchmarking heterogeneous electron cryo-microscopy reconstructions.IUCrJ. 2024 Nov 1;11(Pt 6):951-965. doi: 10.1107/S2052252524009321. IUCrJ. 2024. PMID: 39404610 Free PMC article.
-
Differential roles of putative arginine fingers of AAA+ ATPases Rvb1 and Rvb2.bioRxiv [Preprint]. 2024 May 13:2024.05.13.593962. doi: 10.1101/2024.05.13.593962. bioRxiv. 2024. PMID: 38798342 Free PMC article. Preprint.
-
Maturation and Assembly of mTOR Complexes by the HSP90-R2TP-TTT Chaperone System: Molecular Insights and Mechanisms.Subcell Biochem. 2024;104:459-483. doi: 10.1007/978-3-031-58843-3_17. Subcell Biochem. 2024. PMID: 38963496 Review.
References
Publication types
MeSH terms
Substances
Grants and funding
LinkOut - more resources
Full Text Sources
Miscellaneous