The Functional Role of IgA in the IgM/IgA-Enriched Immunoglobulin Preparation Trimodulin
- PMID: 34944644
- PMCID: PMC8698729
- DOI: 10.3390/biomedicines9121828
The Functional Role of IgA in the IgM/IgA-Enriched Immunoglobulin Preparation Trimodulin
Abstract
In comparison to human immunoglobulin (Ig) G, antibodies of IgA class are not well investigated. In line with this, the functional role of the IgA component in IgM/IgA-enriched immunoglobulin preparations is also largely unknown. In recent years, powerful anti-pathogenic and immunomodulatory properties of human serum IgA especially on neutrophil function were unraveled. Therefore, the aim of our work is to investigate functional aspects of the trimodulin IgA component, a new plasma-derived polyvalent immunoglobulin preparation containing ~56% IgG, ~23% IgM and ~21% IgA. The functional role of IgA was investigated by analyzing the interaction of IgA with FcαRI, comparing trimodulin with standard intravenous IgG (IVIG) preparation and investigating Fc receptor (FcR)-dependent functions by excluding IgM-mediated effects. Trimodulin demonstrated potent immunomodulatory, as well as anti-pathogenic effects in our neutrophil model (neutrophil-like HL-60 cells). The IgA component of trimodulin was shown to induce a strong FcαRI-dependent inhibitory immunoreceptor tyrosine-based activation motif (ITAMi) signaling, counteract lipopolysaccharide-induced inflammation and mediate phagocytosis of Staphylococcus aureus. The fine-tuned balance between immunomodulatory and anti-pathogenic effects of trimodulin were shown to be dose-dependent. Summarized, our data demonstrate the functional role of IgA in trimodulin, highlighting the importance of this immunoglobulin class in immunoglobulin therapy.
Keywords: Fc receptors; FcαRI; ITAMi; IVIG; IgA; cytokines; neutrophils; phagocytosis; trimodulin.
Conflict of interest statement
Authors are employees of Biotest AG, Dreieich, Germany. The authors declare that the research was conducted in the absence of any other commercial or financial relationships that could be construed as a potential conflict of interest.
Figures
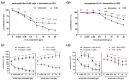
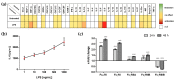
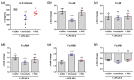
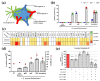
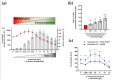
Similar articles
-
The immunomodulating activity of trimodulin (polyvalent IgM, IgA, IgG solution): a post hoc analysis of the phase II CIGMA trial.Crit Care. 2023 Nov 9;27(1):436. doi: 10.1186/s13054-023-04719-9. Crit Care. 2023. PMID: 37946226 Free PMC article. Clinical Trial.
-
Immunomodulation: Immunoglobulin Preparations Suppress Hyperinflammation in a COVID-19 Model via FcγRIIA and FcαRI.Front Immunol. 2021 Jun 10;12:700429. doi: 10.3389/fimmu.2021.700429. eCollection 2021. Front Immunol. 2021. PMID: 34177967 Free PMC article.
-
The Dual Role of a Polyvalent IgM/IgA-Enriched Immunoglobulin Preparation in Activating and Inhibiting the Complement System.Biomedicines. 2021 Jul 14;9(7):817. doi: 10.3390/biomedicines9070817. Biomedicines. 2021. PMID: 34356880 Free PMC article.
-
Anti-inflammatory role of the IgA Fc receptor (CD89): from autoimmunity to therapeutic perspectives.Autoimmun Rev. 2013 Apr;12(6):666-9. doi: 10.1016/j.autrev.2012.10.011. Epub 2012 Nov 29. Autoimmun Rev. 2013. PMID: 23201915 Review.
-
IgA and FcαRI: Pathological Roles and Therapeutic Opportunities.Front Immunol. 2019 Mar 22;10:553. doi: 10.3389/fimmu.2019.00553. eCollection 2019. Front Immunol. 2019. PMID: 30984170 Free PMC article. Review.
Cited by
-
Multifaceted Tissue-Protective Functions of Polyvalent Immunoglobulin Preparations in Severe Infections-Interactions with Neutrophils, Complement, and Coagulation Pathways.Biomedicines. 2023 Nov 10;11(11):3022. doi: 10.3390/biomedicines11113022. Biomedicines. 2023. PMID: 38002022 Free PMC article. Review.
-
Preparation and activity study of Ruoqiang jujube polysaccharide copper chelate.Front Pharmacol. 2024 Jan 11;14:1347817. doi: 10.3389/fphar.2023.1347817. eCollection 2023. Front Pharmacol. 2024. PMID: 38273828 Free PMC article.
-
The immunomodulating activity of trimodulin (polyvalent IgM, IgA, IgG solution): a post hoc analysis of the phase II CIGMA trial.Crit Care. 2023 Nov 9;27(1):436. doi: 10.1186/s13054-023-04719-9. Crit Care. 2023. PMID: 37946226 Free PMC article. Clinical Trial.
-
A new hope? Possibilities of therapeutic IgA antibodies in the treatment of inflammatory lung diseases.Front Immunol. 2023 Mar 27;14:1127339. doi: 10.3389/fimmu.2023.1127339. eCollection 2023. Front Immunol. 2023. PMID: 37051237 Free PMC article. Review.
-
Efficacy and safety of trimodulin in patients with severe COVID-19: results from a randomised, placebo-controlled, double-blind, multicentre, phase II trial (ESsCOVID).Eur J Med Res. 2024 Aug 13;29(1):418. doi: 10.1186/s40001-024-02008-x. Eur J Med Res. 2024. PMID: 39138518 Free PMC article. Clinical Trial.
References
-
- Späth P.J. Structure and Function of Immunoglobulins. Sepsis. 1999;3:197–218. doi: 10.1023/A:1009899803032. - DOI
LinkOut - more resources
Full Text Sources
Miscellaneous