Andrographolide Attenuates Established Pulmonary Hypertension via Rescue of Vascular Remodeling
- PMID: 34944445
- PMCID: PMC8699233
- DOI: 10.3390/biom11121801
Andrographolide Attenuates Established Pulmonary Hypertension via Rescue of Vascular Remodeling
Abstract
Pulmonary hypertension (PH) is characterized by vascular remodeling caused by marked proliferation of pulmonary artery smooth muscle cells (PASMCs). Andrographolide (ANDRO) is a potent anti-inflammatory agent which possesses antioxidant, and has anticarcinogenic activity. The present study examined potential therapeutic effects of ANDRO on PH in both chronic hypoxia and Sugen5416/hypoxia mouse PH models. Effects of ANDRO were also studied in cultured human PASMCs isolated from either healthy donors or PH patients. In vivo, ANDRO decreased distal pulmonary arteries (PAs) remodeling, mean PA pressure and right ventricular hypertrophy in chronic hypoxia- and Sugen/hypoxia-induced PH in mice. ANDRO reduced cell viability, proliferation and migration, but increased cell apoptosis in the PASMCs isolated from PH patients. ANDRO also reversed the dysfunctional bone morphogenetic protein receptor type-2 (BMPR2) signaling, suppressed [Ca2+]i elevation, reactive oxygen species (ROS) generation, and the upregulated expression of IL-6 and IL-8, ET-1 and VEGF in PASMCs from PH patients. Moreover, ANDRO significantly attenuated the activation of TLR4/NF-κB, ERK- and JNK-MAPK signaling pathways and reversed the inhibition of p38-MAPK in PASMCs of PH patients. Further, ANDRO blocked hypoxia-triggered ROS generation by suppressing NADPH oxidase (NOX) activation and augmenting nuclear factor erythroid 2-related factor 2 (Nrf2) expression both in vitro and in vivo. Conventional pulmonary vasodilators have limited efficacy for the treatment of severe PH. We demonstrated that ANDRO may reverse pulmonary vascular remodeling through modulation of NOX/Nrf2-mediated oxidative stress and NF-κB-mediated inflammation. Our findings suggest that ANDRO may have therapeutic value in the treatment of PH.
Keywords: andrographolide; pulmonary artery smooth muscle cells; pulmonary hypertension; vascular remodeling.
Conflict of interest statement
The authors declare no conflict of interest.
Figures
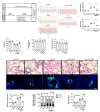
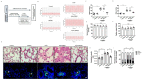
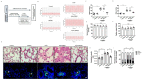
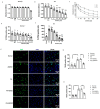
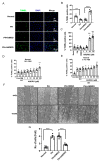
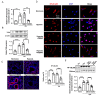
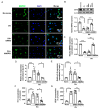
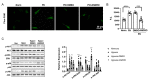
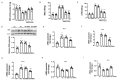
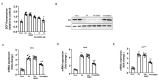
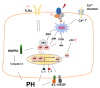
Similar articles
-
Parthenolide attenuates hypoxia-induced pulmonary hypertension through inhibiting STAT3 signaling.Phytomedicine. 2024 Nov;134:155976. doi: 10.1016/j.phymed.2024.155976. Epub 2024 Aug 22. Phytomedicine. 2024. PMID: 39265445
-
TRB3 mediates vascular remodeling by activating the MAPK signaling pathway in hypoxic pulmonary hypertension.Respir Res. 2021 Dec 14;22(1):312. doi: 10.1186/s12931-021-01908-4. Respir Res. 2021. PMID: 34906150 Free PMC article.
-
Critical role for the advanced glycation end-products receptor in pulmonary arterial hypertension etiology.J Am Heart Assoc. 2013 Jan 16;2(1):e005157. doi: 10.1161/JAHA.112.005157. J Am Heart Assoc. 2013. PMID: 23525442 Free PMC article.
-
Calcium sensing receptor: A promising therapeutic target in pulmonary hypertension.Life Sci. 2024 Mar 1;340:122472. doi: 10.1016/j.lfs.2024.122472. Epub 2024 Jan 28. Life Sci. 2024. PMID: 38290572 Review.
-
Novel Targets of Drug Treatment for Pulmonary Hypertension.Am J Cardiovasc Drugs. 2015 Aug;15(4):225-34. doi: 10.1007/s40256-015-0125-4. Am J Cardiovasc Drugs. 2015. PMID: 26016608 Review.
Cited by
-
Nrf2-Mediated Dichotomy in the Vascular System: Mechanistic and Therapeutic Perspective.Cells. 2022 Sep 28;11(19):3042. doi: 10.3390/cells11193042. Cells. 2022. PMID: 36231004 Free PMC article. Review.
-
4-hydroxysesamin protects rat with right ventricular failure due to pulmonary hypertension by inhibiting JNK/p38 MAPK signaling.Aging (Albany NY). 2024 May 8;16(9):8142-8154. doi: 10.18632/aging.205808. Epub 2024 May 8. Aging (Albany NY). 2024. PMID: 38728253 Free PMC article.
-
Target Nuclear Factor Erythroid 2-Related Factor 2 in Pulmonary Hypertension: Molecular Insight into Application.Oxid Med Cell Longev. 2022 Jun 6;2022:7845503. doi: 10.1155/2022/7845503. eCollection 2022. Oxid Med Cell Longev. 2022. PMID: 35707273 Free PMC article. Review.
-
Interleukin-6 and pulmonary hypertension: from physiopathology to therapy.Front Immunol. 2023 Jun 28;14:1181987. doi: 10.3389/fimmu.2023.1181987. eCollection 2023. Front Immunol. 2023. PMID: 37449201 Free PMC article. Review.
-
Cell Death in Pulmonary Arterial Hypertension.Int J Med Sci. 2024 Jul 14;21(10):1840-1851. doi: 10.7150/ijms.93902. eCollection 2024. Int J Med Sci. 2024. PMID: 39113898 Free PMC article. Review.
References
-
- Gomberg-Maitland M., Maitland M.L., Barst R.J., Sugeng L., Coslet S., Perrino T.J., Bond L., Lacouture M.E., Archer S.L., Ratain M.J. A dosing/cross-development study of the multikinase inhibitor sorafenib in patients with pulmonary arterial hypertension. Clin. Pharmacol. Ther. 2010;87:303–310. doi: 10.1038/clpt.2009.217. - DOI - PMC - PubMed
Publication types
MeSH terms
Substances
LinkOut - more resources
Full Text Sources
Medical
Research Materials
Miscellaneous