Mechanistic diversity in MHC class I antigen recognition
- PMID: 34940832
- PMCID: PMC8786304
- DOI: 10.1042/BCJ20200910
Mechanistic diversity in MHC class I antigen recognition
Abstract
Throughout its evolution, the human immune system has developed a plethora of strategies to diversify the antigenic peptide sequences that can be targeted by the CD8+ T cell response against pathogens and aberrations of self. Here we provide a general overview of the mechanisms that lead to the diversity of antigens presented by MHC class I complexes and their recognition by CD8+ T cells, together with a more detailed analysis of recent progress in two important areas that are highly controversial: the prevalence and immunological relevance of unconventional antigen peptides; and cross-recognition of antigenic peptides by the T cell receptors of CD8+ T cells.
Keywords: MHC class I; T cells; TCR; antigens; spliced peptides.
© 2021 The Author(s).
Conflict of interest statement
The authors declare that there are no competing interests associated with the manuscript.
Figures
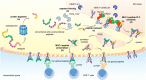
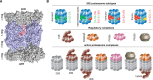
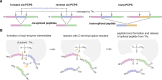
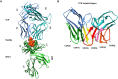
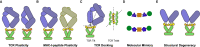
Similar articles
-
Impact of CD8-MHC class I interaction in detection and sorting efficiencies of antigen-specific T cells using MHC class I/peptide multimers: contribution of pMHC valency.Int Immunol. 2006 Jul;18(7):1139-45. doi: 10.1093/intimm/dxl048. Epub 2006 Jun 2. Int Immunol. 2006. PMID: 16751231
-
Soluble peptide-MHC monomers cause activation of CD8+ T cells through transfer of the peptide to T cell MHC molecules.Proc Natl Acad Sci U S A. 2002 Oct 15;99(21):13729-34. doi: 10.1073/pnas.212515299. Epub 2002 Oct 8. Proc Natl Acad Sci U S A. 2002. PMID: 12374859 Free PMC article.
-
CD8 coreceptor-mediated focusing can reorder the agonist hierarchy of peptide ligands recognized via the T cell receptor.Proc Natl Acad Sci U S A. 2021 Jul 20;118(29):e2019639118. doi: 10.1073/pnas.2019639118. Proc Natl Acad Sci U S A. 2021. PMID: 34272276 Free PMC article.
-
TCR Recognition of Peptide-MHC-I: Rule Makers and Breakers.Int J Mol Sci. 2020 Dec 23;22(1):68. doi: 10.3390/ijms22010068. Int J Mol Sci. 2020. PMID: 33374673 Free PMC article. Review.
-
Recognition surfaces of MHC class I.Immunol Rev. 1998 Jun;163:121-8. doi: 10.1111/j.1600-065x.1998.tb01191.x. Immunol Rev. 1998. PMID: 9700505 Review.
Cited by
-
β2-microglobulin and colorectal cancer among inpatients: a case-control study.Sci Rep. 2023 Jul 27;13(1):12222. doi: 10.1038/s41598-023-39162-x. Sci Rep. 2023. PMID: 37500738 Free PMC article.
-
Immunotherapy: A new target for cancer cure (Review).Oncol Rep. 2023 May;49(5):100. doi: 10.3892/or.2023.8537. Epub 2023 Mar 31. Oncol Rep. 2023. PMID: 36999633 Free PMC article. Review.
-
Protein degradation by human 20S proteasomes elucidates the interplay between peptide hydrolysis and splicing.Nat Commun. 2024 Feb 7;15(1):1147. doi: 10.1038/s41467-024-45339-3. Nat Commun. 2024. PMID: 38326304 Free PMC article.
-
Database search engines and target database features impinge upon the identification of post-translationally cis-spliced peptides in HLA class I immunopeptidomes.Proteomics. 2022 May;22(10):e2100226. doi: 10.1002/pmic.202100226. Epub 2022 Mar 3. Proteomics. 2022. PMID: 35184383 Free PMC article.
-
iBench: A ground truth approach for advanced validation of mass spectrometry identification method.Proteomics. 2023 Jan;23(2):e2200271. doi: 10.1002/pmic.202200271. Epub 2022 Oct 17. Proteomics. 2023. PMID: 36189881 Free PMC article.
References
-
- Janeway, C.A., Travers, P., Walport, M. and Shlomchik, M.J. (2001) T cell-mediated cytotoxicity. In Immunobiology: The Immune System in Health and Disease, Garland Science, New York
Publication types
MeSH terms
Substances
Grants and funding
LinkOut - more resources
Full Text Sources
Research Materials