Current hydrogel advances in physicochemical and biological response-driven biomedical application diversity
- PMID: 34916490
- PMCID: PMC8674418
- DOI: 10.1038/s41392-021-00830-x
Current hydrogel advances in physicochemical and biological response-driven biomedical application diversity
Abstract
Hydrogel is a type of versatile platform with various biomedical applications after rational structure and functional design that leverages on material engineering to modulate its physicochemical properties (e.g., stiffness, pore size, viscoelasticity, microarchitecture, degradability, ligand presentation, stimulus-responsive properties, etc.) and influence cell signaling cascades and fate. In the past few decades, a plethora of pioneering studies have been implemented to explore the cell-hydrogel matrix interactions and figure out the underlying mechanisms, paving the way to the lab-to-clinic translation of hydrogel-based therapies. In this review, we first introduced the physicochemical properties of hydrogels and their fabrication approaches concisely. Subsequently, the comprehensive description and deep discussion were elucidated, wherein the influences of different hydrogels properties on cell behaviors and cellular signaling events were highlighted. These behaviors or events included integrin clustering, focal adhesion (FA) complex accumulation and activation, cytoskeleton rearrangement, protein cyto-nuclei shuttling and activation (e.g., Yes-associated protein (YAP), catenin, etc.), cellular compartment reorganization, gene expression, and further cell biology modulation (e.g., spreading, migration, proliferation, lineage commitment, etc.). Based on them, current in vitro and in vivo hydrogel applications that mainly covered diseases models, various cell delivery protocols for tissue regeneration and disease therapy, smart drug carrier, bioimaging, biosensor, and conductive wearable/implantable biodevices, etc. were further summarized and discussed. More significantly, the clinical translation potential and trials of hydrogels were presented, accompanied with which the remaining challenges and future perspectives in this field were emphasized. Collectively, the comprehensive and deep insights in this review will shed light on the design principles of new biomedical hydrogels to understand and modulate cellular processes, which are available for providing significant indications for future hydrogel design and serving for a broad range of biomedical applications.
© 2021. The Author(s).
Conflict of interest statement
The authors declare no competing interests.
Figures
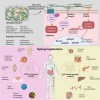
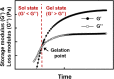
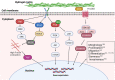
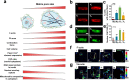
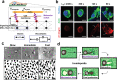
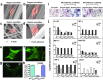
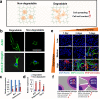
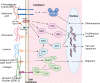
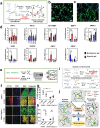
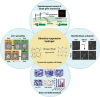
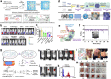
Similar articles
-
Programmable Morphing Hydrogels for Soft Actuators and Robots: From Structure Designs to Active Functions.Acc Chem Res. 2022 Jun 7;55(11):1533-1545. doi: 10.1021/acs.accounts.2c00046. Epub 2022 Apr 12. Acc Chem Res. 2022. PMID: 35413187
-
Self-Assembling Peptide Nanofibrous Hydrogel as a Versatile Drug Delivery Platform.Curr Pharm Des. 2015;21(29):4342-54. doi: 10.2174/1381612821666150901104821. Curr Pharm Des. 2015. PMID: 26323419 Review.
-
High-Performance Smart Hydrogels with Redox-Responsive Properties Inspired by Scallop Byssus.ACS Appl Mater Interfaces. 2022 Jan 12;14(1):214-224. doi: 10.1021/acsami.1c18610. Epub 2021 Dec 22. ACS Appl Mater Interfaces. 2022. PMID: 34935338
-
Interplay between degradability and integrin signaling on mesenchymal stem cell function within poly(ethylene glycol) based microporous annealed particle hydrogels.Acta Biomater. 2020 Jan 1;101:227-236. doi: 10.1016/j.actbio.2019.11.009. Epub 2019 Nov 8. Acta Biomater. 2020. PMID: 31711899 Free PMC article.
-
Engineered Hydrogels in Cancer Therapy and Diagnosis.Trends Biotechnol. 2017 Nov;35(11):1074-1087. doi: 10.1016/j.tibtech.2017.06.015. Epub 2017 Jul 19. Trends Biotechnol. 2017. PMID: 28734545 Review.
Cited by
-
Harnessing the potential of hydrogels for advanced therapeutic applications: current achievements and future directions.Signal Transduct Target Ther. 2024 Jul 1;9(1):166. doi: 10.1038/s41392-024-01852-x. Signal Transduct Target Ther. 2024. PMID: 38945949 Free PMC article. Review.
-
Tertiary lymphoid structures in diseases: immune mechanisms and therapeutic advances.Signal Transduct Target Ther. 2024 Aug 28;9(1):225. doi: 10.1038/s41392-024-01947-5. Signal Transduct Target Ther. 2024. PMID: 39198425 Free PMC article. Review.
-
Modulation of Methacrylated Hyaluronic Acid Hydrogels Enables Their Use as 3D Cultured Model.Gels. 2023 Oct 5;9(10):801. doi: 10.3390/gels9100801. Gels. 2023. PMID: 37888374 Free PMC article.
-
Hydrogels for Cardiac Restorative Support: Relevance of Gelation Mechanisms for Prospective Clinical Use.Curr Heart Fail Rep. 2023 Dec;20(6):519-529. doi: 10.1007/s11897-023-00630-0. Epub 2023 Oct 9. Curr Heart Fail Rep. 2023. PMID: 37812347 Free PMC article. Review.
-
Building Fucoidan/Agarose-Based Hydrogels as a Platform for the Development of Therapeutic Approaches against Diabetes.Molecules. 2023 Jun 2;28(11):4523. doi: 10.3390/molecules28114523. Molecules. 2023. PMID: 37298999 Free PMC article.
References
-
- Lin W, et al. Cartilage-inspired, lipid-based boundary-lubricated hydrogels. Science. 2020;370:335–338. - PubMed
-
- Contessotto P, et al. Elastin-like recombinamers-based hydrogel modulates post-ischemic remodeling in a non-transmural myocardial infarction in sheep. Sci. Transl. Med. 2021;13:eaaz5380. - PubMed
-
- Hogrebe NJ, Reinhardt JW, Gooch KJ. Biomaterial microarchitecture: a potent regulator of individual cell behavior and multicellular organization. J. Biomed. Mater. Res. A. 2017;105:640–661. - PubMed
Publication types
MeSH terms
Substances
LinkOut - more resources
Full Text Sources
Miscellaneous