Mitochondria in Early Forebrain Development: From Neurulation to Mid-Corticogenesis
- PMID: 34888312
- PMCID: PMC8650308
- DOI: 10.3389/fcell.2021.780207
Mitochondria in Early Forebrain Development: From Neurulation to Mid-Corticogenesis
Abstract
Function of the mature central nervous system (CNS) requires a substantial proportion of the body's energy consumption. During development, the CNS anlage must maintain its structure and perform stage-specific functions as it proceeds through discrete developmental stages. While key extrinsic signals and internal transcriptional controls over these processes are well appreciated, metabolic and mitochondrial states are also critical to appropriate forebrain development. Specifically, metabolic state, mitochondrial function, and mitochondrial dynamics/localization play critical roles in neurulation and CNS progenitor specification, progenitor proliferation and survival, neurogenesis, neural migration, and neurite outgrowth and synaptogenesis. With the goal of integrating neurodevelopmental biologists and mitochondrial specialists, this review synthesizes data from disparate models and processes to compile and highlight key roles of mitochondria in the early development of the CNS with specific focus on forebrain development and corticogenesis.
Keywords: corticogenesis; development; forebrain; metabolism; mitochondria; neural tube closure; neurulation.
Copyright © 2021 Fame and Lehtinen.
Conflict of interest statement
The authors declare that the research was conducted in the absence of any commercial or financial relationships that could be construed as a potential conflict of interest.
Figures
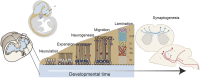
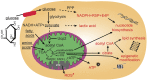
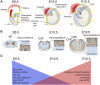
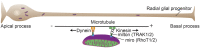
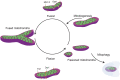
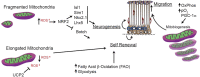
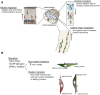
Similar articles
-
Brain development and bioenergetic changes.Neurobiol Dis. 2024 Sep;199:106550. doi: 10.1016/j.nbd.2024.106550. Epub 2024 Jun 6. Neurobiol Dis. 2024. PMID: 38849103 Free PMC article. Review.
-
The Neocortical Progenitor Specification Program Is Established through Combined Modulation of SHH and FGF Signaling.J Neurosci. 2020 Sep 2;40(36):6872-6887. doi: 10.1523/JNEUROSCI.2888-19.2020. Epub 2020 Jul 31. J Neurosci. 2020. PMID: 32737167 Free PMC article.
-
Sequence and developmental expression of AmphiDll, an amphioxus Distal-less gene transcribed in the ectoderm, epidermis and nervous system: insights into evolution of craniate forebrain and neural crest.Development. 1996 Sep;122(9):2911-20. doi: 10.1242/dev.122.9.2911. Development. 1996. PMID: 8787764
-
A concerted metabolic shift in early forebrain alters the CSF proteome and depends on MYC downregulation for mitochondrial maturation.Development. 2019 Oct 24;146(20):dev182857. doi: 10.1242/dev.182857. Development. 2019. PMID: 31575649 Free PMC article.
-
Transcriptional and epigenetic regulation of neural crest induction during neurulation.Dev Neurosci. 2013;35(5):361-72. doi: 10.1159/000354749. Epub 2013 Sep 19. Dev Neurosci. 2013. PMID: 24051984 Review.
Cited by
-
Dysregulation of FLVCR1a-dependent mitochondrial calcium handling in neural progenitors causes congenital hydrocephalus.Cell Rep Med. 2024 Jul 16;5(7):101647. doi: 10.1016/j.xcrm.2024.101647. Cell Rep Med. 2024. PMID: 39019006 Free PMC article.
-
Analysis of mitochondrial respiration and ATP synthase in frozen brain tissues.Heliyon. 2023 Feb 22;9(3):e13888. doi: 10.1016/j.heliyon.2023.e13888. eCollection 2023 Mar. Heliyon. 2023. PMID: 36895388 Free PMC article.
-
Pain/Stress, Mitochondrial Dysfunction, and Neurodevelopment in Preterm Infants.Dev Neurosci. 2024;46(5):341-352. doi: 10.1159/000536509. Epub 2024 Jan 29. Dev Neurosci. 2024. PMID: 38286121 Free PMC article.
-
Brain development and bioenergetic changes.Neurobiol Dis. 2024 Sep;199:106550. doi: 10.1016/j.nbd.2024.106550. Epub 2024 Jun 6. Neurobiol Dis. 2024. PMID: 38849103 Free PMC article. Review.
-
Mitochondria play an essential role in the trajectory of adolescent neurodevelopment and behavior in adulthood: evidence from a schizophrenia rat model.Mol Psychiatry. 2023 Mar;28(3):1170-1181. doi: 10.1038/s41380-022-01865-4. Epub 2022 Nov 15. Mol Psychiatry. 2023. PMID: 36380234 Free PMC article.
References
Publication types
Grants and funding
LinkOut - more resources
Full Text Sources