Perfluorinated Carboxylic Acids with Increasing Carbon Chain Lengths Upregulate Amino Acid Transporters and Modulate Compensatory Response of Xenobiotic Transporters in HepaRG Cells
- PMID: 34857530
- PMCID: PMC9513853
- DOI: 10.1124/dmd.121.000477
Perfluorinated Carboxylic Acids with Increasing Carbon Chain Lengths Upregulate Amino Acid Transporters and Modulate Compensatory Response of Xenobiotic Transporters in HepaRG Cells
Abstract
Perfluorinated carboxylic acids (PFCAs) are widespread environmental pollutants for which human exposure has been documented. PFCAs at high doses are known to regulate xenobiotic transporters partly through peroxisome proliferator-activated receptor alpha (PPARα) and constitutive androstane receptor (CAR) in rodent models. Less is known regarding how various PFCAs at a lower concentration modulate transporters for endogenous substrates, such as amino acids in human hepatocytes. Such studies are of particular importance because amino acids are involved in chemical detoxification, and their transport system may serve as a promising therapeutic target for structurally similar xenobiotics. The focus of this study was to further elucidate how PFCAs modulate transporters involved in intermediary metabolism and xenobiotic biotransformation. We tested the hepatic transcriptomic response of HepaRG cells exposed to 45 μM of perfluorooctanoic acid, perfluorononanoic acid, or perfluorodecanoic acid in triplicates for 24 hours (vehicle: 0.1% DMSO), as well as the prototypical ligands for PPARα (WY-14643, 45 μM) and CAR (6-(4-chlorophenyl)imidazo[2,1-b][1,3]thiazole-5-carbaldehyde O-(3,4-dichlorobenzyl)oxime [CITCO], 2 μM). PFCAs with increasing carbon chain lengths (C8-C10) regulated more liver genes, with amino acid metabolism and transport ranked among the top enriched pathways and PFDA ranked as the most potent PFCA tested. Genes encoding amino acid transporters, which are essential for protein synthesis, were novel inducible targets by all three PFCAs, suggesting a potentially protective mechanism to reduce further toxic insults. None of the transporter regulations appeared to be through PPARα or CAR but potential involvement of nuclear factor erythroid 2-related factor 2 is noted for all 3 PFCAs. In conclusion, PFCAs with increasing carbon chain lengths up-regulate amino acid transporters and modulate xenobiotic transporters to limit further toxic exposures in HepaRG cells. SIGNIFICANCE STATEMENT: Little is known regarding how various perfluorinated carboxylic acids modulate the transporters for endogenous substrates in human liver cells. Using HepaRG cells, this study is among the first to show that perfluorinated carboxylic acids with increasing carbon chain lengths upregulate amino acid transporters, which are essential for protein synthesis, and modulate xenobiotic transporters to limit further toxic exposures at concentrations lower than what was used in the literature.
Copyright © 2022 by The American Society for Pharmacology and Experimental Therapeutics.
Figures
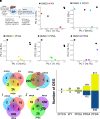
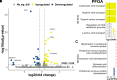
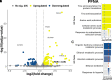
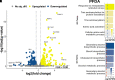
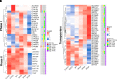
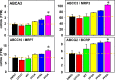
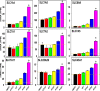
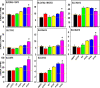
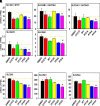
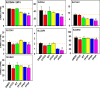
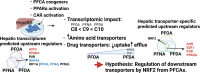
Similar articles
-
Activation of nuclear receptor CAR by an environmental pollutant perfluorooctanoic acid.Arch Toxicol. 2017 Jun;91(6):2365-2374. doi: 10.1007/s00204-016-1888-3. Epub 2016 Nov 10. Arch Toxicol. 2017. PMID: 27832320
-
Activation of mouse and human peroxisome proliferator-activated receptor alpha by perfluoroalkyl acids of different functional groups and chain lengths.Toxicol Sci. 2008 Nov;106(1):162-71. doi: 10.1093/toxsci/kfn166. Epub 2008 Aug 18. Toxicol Sci. 2008. PMID: 18713766
-
Perfluorocarboxylic acids induce cytochrome P450 enzymes in mouse liver through activation of PPAR-alpha and CAR transcription factors.Toxicol Sci. 2008 Nov;106(1):29-36. doi: 10.1093/toxsci/kfn147. Epub 2008 Jul 22. Toxicol Sci. 2008. PMID: 18648086 Free PMC article.
-
Role of hepatic fatty acid:coenzyme A ligases in the metabolism of xenobiotic carboxylic acids.Clin Exp Pharmacol Physiol. 1998 Oct;25(10):776-82. doi: 10.1111/j.1440-1681.1998.tb02152.x. Clin Exp Pharmacol Physiol. 1998. PMID: 9784915 Review.
-
Induction of phase I, II and III drug metabolism/transport by xenobiotics.Arch Pharm Res. 2005 Mar;28(3):249-68. doi: 10.1007/BF02977789. Arch Pharm Res. 2005. PMID: 15832810 Review.
Cited by
-
Effects of PFAS on human liver transporters: implications for health outcomes.Toxicol Sci. 2024 Aug 1;200(2):213-227. doi: 10.1093/toxsci/kfae061. Toxicol Sci. 2024. PMID: 38724241 Free PMC article. Review.
References
-
- Alexa A, Rahnenfuhrer J (2021) topGO: Enrichment Analysis for Gene Ontology. R package version 2.44.0.
-
- Abe TTakahashi MKano MAmaike YIshii CMaeda KKudoh YMorishita THosaka TSasaki T, et al. (2017) Activation of nuclear receptor CAR by an environmental pollutant perfluorooctanoic acid. Arch Toxicol 91:2365–2374. - PubMed
-
- Boisvert G, Sonne C, Rigét FF, Dietz R, Letcher RJ (2019) Bioaccumulation and biomagnification of perfluoroalkyl acids and precursors in East Greenland polar bears and their ringed seal prey. Environ Pollut 252 (Pt B):1335–1343. - PubMed
Publication types
MeSH terms
Substances
Grants and funding
LinkOut - more resources
Full Text Sources