Mutagenesis of the Varicella-Zoster Virus Genome Demonstrates That VLT and VLT-ORF63 Proteins Are Dispensable for Lytic Infection
- PMID: 34835095
- PMCID: PMC8619377
- DOI: 10.3390/v13112289
Mutagenesis of the Varicella-Zoster Virus Genome Demonstrates That VLT and VLT-ORF63 Proteins Are Dispensable for Lytic Infection
Abstract
Primary varicella-zoster virus (VZV) infection leads to varicella and the establishment of lifelong latency in sensory ganglion neurons. Reactivation of latent VZV causes herpes zoster, which is frequently associated with chronic pain. Latent viral gene expression is restricted to the VZV latency-associated transcript (VLT) and VLT-ORF63 (VLT63) fusion transcripts. Since VLT and VLT63 encode proteins that are expressed during lytic infection, we investigated whether pVLT and pVLT-ORF63 are essential for VZV replication by performing VZV genome mutagenesis using CRISPR/Cas9 and BAC technologies. We first established that CRISPR/Cas9 can efficiently mutate VZV genomes in lytically VZV-infected cells through targeting non-essential genes ORF8 and ORF11 and subsequently show recovery of viable mutant viruses. By contrast, the VLT region was markedly resistant to CRISPR/Cas9 editing. Whereas most mutants expressed wild-type or N-terminally altered versions of pVLT and pVLT-ORF63, only a minority of the resulting mutant viruses lacked pVLT and pVLT-ORF63 coding potential. Growth curve analysis showed that pVLT/pVLT-ORF63 negative viruses were viable, but impaired in growth in epithelial cells. We confirmed this phenotype independently using BAC-derived pVLT/pVLT-ORF63 negative and repaired viruses. Collectively, these data demonstrate that pVLT and/or pVLT-ORF63 are dispensable for lytic VZV replication but promote efficient VZV infection in epithelial cells.
Keywords: BAC mutagenesis; CRISPR/Cas9; VLT; VLT-ORF63; varicella-zoster virus.
Conflict of interest statement
The authors declare no conflict of interest.
Figures
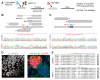
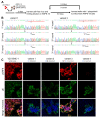
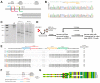
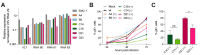
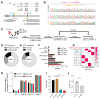
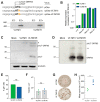
Similar articles
-
Varicella-zoster virus VLT-ORF63 fusion transcript induces broad viral gene expression during reactivation from neuronal latency.Nat Commun. 2020 Dec 10;11(1):6324. doi: 10.1038/s41467-020-20031-4. Nat Commun. 2020. PMID: 33303747 Free PMC article.
-
Downregulation of varicella-zoster virus (VZV) immediate-early ORF62 transcription by VZV ORF63 correlates with virus replication in vitro and with latency.J Virol. 2006 Apr;80(7):3459-68. doi: 10.1128/JVI.80.7.3459-3468.2006. J Virol. 2006. PMID: 16537613 Free PMC article.
-
Regions of the varicella-zoster virus open reading frame 63 latency-associated protein important for replication in vitro are also critical for efficient establishment of latency.J Virol. 2005 Apr;79(8):5069-77. doi: 10.1128/JVI.79.8.5069-5077.2005. J Virol. 2005. PMID: 15795292 Free PMC article.
-
A comparison of herpes simplex virus type 1 and varicella-zoster virus latency and reactivation.J Gen Virol. 2015 Jul;96(Pt 7):1581-602. doi: 10.1099/vir.0.000128. Epub 2015 Mar 20. J Gen Virol. 2015. PMID: 25794504 Free PMC article. Review.
-
Molecular Aspects of Varicella-Zoster Virus Latency.Viruses. 2018 Jun 28;10(7):349. doi: 10.3390/v10070349. Viruses. 2018. PMID: 29958408 Free PMC article. Review.
Cited by
-
Antiviral Targeting of Varicella Zoster Virus Replication and Neuronal Reactivation Using CRISPR/Cas9 Cleavage of the Duplicated Open Reading Frames 62/71.Viruses. 2022 Feb 12;14(2):378. doi: 10.3390/v14020378. Viruses. 2022. PMID: 35215971 Free PMC article.
-
Fraternal Twins: The Enigmatic Role of the Immune System in Alphaherpesvirus Pathogenesis and Latency and Its Impacts on Vaccine Efficacy.Viruses. 2022 Apr 21;14(5):862. doi: 10.3390/v14050862. Viruses. 2022. PMID: 35632603 Free PMC article. Review.
References
Publication types
MeSH terms
Substances
Grants and funding
LinkOut - more resources
Full Text Sources