Development and Adoption of Genetically Engineered Plants for Virus Resistance: Advances, Opportunities and Challenges
- PMID: 34834702
- PMCID: PMC8623320
- DOI: 10.3390/plants10112339
Development and Adoption of Genetically Engineered Plants for Virus Resistance: Advances, Opportunities and Challenges
Abstract
Plant viruses cause yield losses to crops of agronomic and economic significance and are a challenge to the achievement of global food security. Although conventional plant breeding has played an important role in managing plant viral diseases, it will unlikely meet the challenges posed by the frequent emergence of novel and more virulent viral species or viral strains. Hence there is an urgent need to seek alternative strategies of virus control that can be more readily deployed to contain viral diseases. The discovery in the late 1980s that viral genes can be introduced into plants to engineer resistance to the cognate virus provided a new avenue for virus disease control. Subsequent advances in genomics and biotechnology have led to the refinement and expansion of genetic engineering (GE) strategies in crop improvement. Importantly, many of the drawbacks of conventional breeding, such as long lead times, inability or difficulty to cross fertilize, loss of desirable plant traits, are overcome by GE. Unfortunately, public skepticism towards genetically modified (GM) crops and other factors have dampened the early promise of GE efforts. These concerns are principally about the possible negative effects of transgenes to humans and animals, as well as to the environment. However, with regards to engineering for virus resistance, these risks are overstated given that most virus resistance engineering strategies involve transfer of viral genes or genomic segments to plants. These viral genomes are found in infected plant cells and have not been associated with any adverse effects in humans or animals. Thus, integrating antiviral genes of virus origin into plant genomes is hardly unnatural as suggested by GM crop skeptics. Moreover, advances in deep sequencing have resulted in the sequencing of large numbers of plant genomes and the revelation of widespread endogenization of viral genomes into plant genomes. This has raised the possibility that viral genome endogenization is part of an antiviral defense mechanism deployed by the plant during its evolutionary past. Thus, GM crops engineered for viral resistance would likely be acceptable to the public if regulatory policies were product-based (the North America regulatory model), as opposed to process-based. This review discusses some of the benefits to be gained from adopting GE for virus resistance, as well as the challenges that must be overcome to leverage this technology. Furthermore, regulatory policies impacting virus-resistant GM crops and some success cases of virus-resistant GM crops approved so far for cultivation are discussed.
Keywords: genetically engineered (GE); genetically engineered organism (GMO); regulation of GMOs; virus-resistant transgenic crops.
Conflict of interest statement
The authors declare no conflict of interest.
Figures
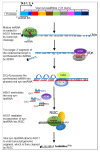
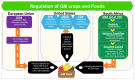
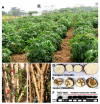
Similar articles
-
Genetically modified (GM) crops: milestones and new advances in crop improvement.Theor Appl Genet. 2016 Sep;129(9):1639-55. doi: 10.1007/s00122-016-2747-6. Epub 2016 Jul 5. Theor Appl Genet. 2016. PMID: 27381849 Review.
-
Lessons learned from the introduction of genetically engineered crops: relevance to gene drive deployment in Africa.Transgenic Res. 2022 Jun;31(3):285-311. doi: 10.1007/s11248-022-00300-2. Epub 2022 May 11. Transgenic Res. 2022. PMID: 35545692 Free PMC article. Review.
-
Genetically engineered virus-resistant plants in developing countries: current status and future prospects.Adv Virus Res. 2009;75:185-220. doi: 10.1016/S0065-3527(09)07506-X. Epub 2010 Jan 13. Adv Virus Res. 2009. PMID: 20109667 Review.
-
Genetically modified crops: current status and future prospects.Planta. 2020 Mar 31;251(4):91. doi: 10.1007/s00425-020-03372-8. Planta. 2020. PMID: 32236850 Review.
-
Next biotech plants: new traits, crops, developers and technologies for addressing global challenges.Crit Rev Biotechnol. 2016 Aug;36(4):675-90. doi: 10.3109/07388551.2015.1004521. Epub 2015 Feb 2. Crit Rev Biotechnol. 2016. PMID: 25641327 Review.
Cited by
-
Virus-Induced Plant Volatiles Promote Virus Acquisition and Transmission by Insect Vectors.Int J Mol Sci. 2023 Jan 16;24(2):1777. doi: 10.3390/ijms24021777. Int J Mol Sci. 2023. PMID: 36675290 Free PMC article.
-
Biotechnologically Engineered Plants.Biology (Basel). 2023 Apr 15;12(4):601. doi: 10.3390/biology12040601. Biology (Basel). 2023. PMID: 37106801 Free PMC article. Review.
-
An atlas of small RNAs from potato.Plant Direct. 2022 Dec 14;6(12):e466. doi: 10.1002/pld3.466. eCollection 2022 Dec. Plant Direct. 2022. PMID: 36530592 Free PMC article.
-
Molecular markers associated with resistance to squash leaf curl China virus and tomato leaf curl New Delhi virus in tropical pumpkin (Cucurbita moschata Duchesne ex Poir.) breeding line AVPU1426.Sci Rep. 2024 Mar 21;14(1):6793. doi: 10.1038/s41598-024-57348-9. Sci Rep. 2024. PMID: 38514827 Free PMC article.
-
[Developmental toxicity of Cry1Ab protein in the embryonic stem-cell model].Beijing Da Xue Xue Bao Yi Xue Ban. 2024 Apr 18;56(2):213-222. doi: 10.19723/j.issn.1671-167X.2024.02.003. Beijing Da Xue Xue Bao Yi Xue Ban. 2024. PMID: 38595236 Free PMC article. Chinese.
References
-
- Patil B.L. Plant viral diseases: Economic implications. In: Bamford D.H., Zuckerman M., editors. Encyclopedia of Virology. 4th ed. Academic Press; Oxford, UK: 2021. pp. 81–97.
-
- Bevan M.W., Flavell R.B., Chilton M.D. A Chimaeric Antibiotic Resistance Gene as a Selectable Marker for Plant Cell Transformation. 1983. Biotechnology. 1992;24:367–370. - PubMed
Publication types
Grants and funding
LinkOut - more resources
Full Text Sources
Miscellaneous