Microtubules regulate pancreatic β-cell heterogeneity via spatiotemporal control of insulin secretion hot spots
- PMID: 34783306
- PMCID: PMC8635970
- DOI: 10.7554/eLife.59912
Microtubules regulate pancreatic β-cell heterogeneity via spatiotemporal control of insulin secretion hot spots
Abstract
Heterogeneity of glucose-stimulated insulin secretion (GSIS) in pancreatic islets is physiologically important but poorly understood. Here, we utilize mouse islets to determine how microtubules (MTs) affect secretion toward the vascular extracellular matrix at single cell and subcellular levels. Our data indicate that MT stability in the β-cell population is heterogenous, and that GSIS is suppressed in cells with highly stable MTs. Consistently, MT hyper-stabilization prevents, and MT depolymerization promotes the capacity of single β-cell for GSIS. Analysis of spatiotemporal patterns of secretion events shows that MT depolymerization activates otherwise dormant β-cells via initiation of secretion clusters (hot spots). MT depolymerization also enhances secretion from individual cells, introducing both additional clusters and scattered events. Interestingly, without MTs, the timing of clustered secretion is dysregulated, extending the first phase of GSIS and causing oversecretion. In contrast, glucose-induced Ca2+ influx was not affected by MT depolymerization yet required for secretion under these conditions, indicating that MT-dependent regulation of secretion hot spots acts in parallel with Ca2+ signaling. Our findings uncover a novel MT function in tuning insulin secretion hot spots, which leads to accurately measured and timed response to glucose stimuli and promotes functional β-cell heterogeneity.
Keywords: biphasic secretion; cell biology; computational cluster analysis; diabetes; medicine; microtubule dynamics; microtubule stability; mouse; stimulus-secretion coupling.
© 2021, Trogden et al.
Conflict of interest statement
KT, JL, KB, KH, HM, XZ, GA, TF, AO, MM, MZ, GG, WH, IK No competing interests declared
Figures
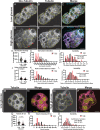
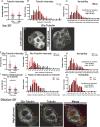
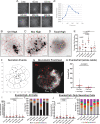
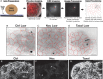
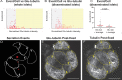
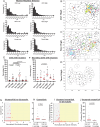
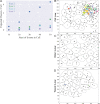
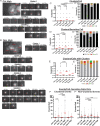
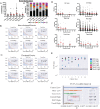
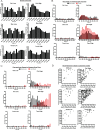
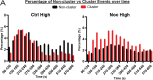
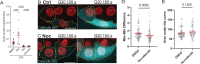
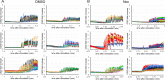
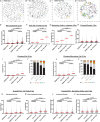
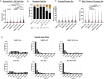
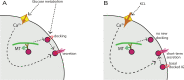
Similar articles
-
Microtubules in Pancreatic β Cells: Convoluted Roadways Toward Precision.Front Cell Dev Biol. 2022 Jul 8;10:915206. doi: 10.3389/fcell.2022.915206. eCollection 2022. Front Cell Dev Biol. 2022. PMID: 35874834 Free PMC article. Review.
-
Microtubules Negatively Regulate Insulin Secretion in Pancreatic β Cells.Dev Cell. 2015 Sep 28;34(6):656-68. doi: 10.1016/j.devcel.2015.08.020. Dev Cell. 2015. PMID: 26418295 Free PMC article.
-
Microtubules Regulate Localization and Availability of Insulin Granules in Pancreatic Beta Cells.Biophys J. 2020 Jan 7;118(1):193-206. doi: 10.1016/j.bpj.2019.10.031. Epub 2019 Oct 31. Biophys J. 2020. PMID: 31839261 Free PMC article.
-
Glucose Regulates Microtubule Disassembly and the Dose of Insulin Secretion via Tau Phosphorylation.Diabetes. 2020 Sep;69(9):1936-1947. doi: 10.2337/db19-1186. Epub 2020 Jun 15. Diabetes. 2020. PMID: 32540877 Free PMC article.
-
Prkar1a in the regulation of insulin secretion.Horm Metab Res. 2012 Sep;44(10):759-65. doi: 10.1055/s-0032-1321866. Epub 2012 Sep 5. Horm Metab Res. 2012. PMID: 22951902 Free PMC article. Review.
Cited by
-
Local activation of focal adhesion kinase orchestrates the positioning of presynaptic scaffold proteins and Ca2+ signalling to control glucose-dependent insulin secretion.Elife. 2022 May 13;11:e76262. doi: 10.7554/eLife.76262. Elife. 2022. PMID: 35559734 Free PMC article.
-
Directed insulin secretion occurs at precise cortical regions with optimal ELKS content that are devoid of microtubules.bioRxiv [Preprint]. 2024 Nov 1:2024.10.31.621333. doi: 10.1101/2024.10.31.621333. bioRxiv. 2024. PMID: 39553950 Free PMC article. Preprint.
-
Unveiling nanoscale optical signatures of cytokine-induced β-cell dysfunction.Sci Rep. 2023 Aug 16;13(1):13342. doi: 10.1038/s41598-023-40272-9. Sci Rep. 2023. PMID: 37587148 Free PMC article.
-
Overcoming the Limitations of Stem Cell-Derived Beta Cells.Biomolecules. 2022 Jun 9;12(6):810. doi: 10.3390/biom12060810. Biomolecules. 2022. PMID: 35740935 Free PMC article. Review.
-
Microtubules in Pancreatic β Cells: Convoluted Roadways Toward Precision.Front Cell Dev Biol. 2022 Jul 8;10:915206. doi: 10.3389/fcell.2022.915206. eCollection 2022. Front Cell Dev Biol. 2022. PMID: 35874834 Free PMC article. Review.
References
-
- Aguayo-Mazzucato C, van Haaren M, Mruk M, Lee TB, Crawford C, Hollister-Lock J, Sullivan BA, Johnson JW, Ebrahimi A, Dreyfuss JM, Van Deursen J, Weir GC, Bonner-Weir S. β Cell Aging Markers Have Heterogeneous Distribution and Are Induced by Insulin Resistance. Cell Metabolism. 2017;25:898–910. doi: 10.1016/j.cmet.2017.03.015. - DOI - PMC - PubMed
-
- Bader E, Migliorini A, Gegg M, Moruzzi N, Gerdes J, Roscioni SS, Bakhti M, Brandl E, Irmler M, Beckers J, Aichler M, Feuchtinger A, Leitzinger C, Zischka H, Wang-Sattler R, Jastroch M, Tschöp M, Machicao F, Staiger H, Häring H-U, Chmelova H, Chouinard JA, Oskolkov N, Korsgren O, Speier S, Lickert H. Identification of proliferative and mature β-cells in the islets of Langerhans. Nature. 2016;535:430–434. doi: 10.1038/nature18624. - DOI - PubMed
Publication types
MeSH terms
Substances
Grants and funding
LinkOut - more resources
Full Text Sources
Other Literature Sources
Miscellaneous