A conserved arginine in NS5 binds genomic 3' stem-loop RNA for primer-independent initiation of flavivirus RNA replication
- PMID: 34759006
- PMCID: PMC8906541
- DOI: 10.1261/rna.078949.121
A conserved arginine in NS5 binds genomic 3' stem-loop RNA for primer-independent initiation of flavivirus RNA replication
Abstract
The commitment to replicate the RNA genome of flaviviruses without a primer involves RNA-protein interactions that have been shown to include the recognition of the stem-loop A (SLA) in the 5' untranslated region (UTR) by the nonstructural protein NS5. We show that DENV2 NS5 arginine 888, located within the carboxy-terminal 18 residues, is completely conserved in all flaviviruses and interacts specifically with the top-loop of 3'SL in the 3'UTR which contains the pentanucleotide 5'-CACAG-3' previously shown to be critical for flavivirus RNA replication. We present virological and biochemical data showing the importance of this Arg 888 in virus viability and de novo initiation of RNA polymerase activity in vitro. Based on our binding studies, we hypothesize that ternary complex formation of NS5 with 3'SL, followed by dimerization, leads to the formation of the de novo initiation complex that could be regulated by the reversible zipping and unzipping of cis-acting RNA elements.
Keywords: Dengue NS5 protein; Dengue virus; Dengue virus 3' stem–loop; de novo initiation; flaviral RNA replication.
© 2022 Wang et al.; Published by Cold Spring Harbor Laboratory Press for the RNA Society.
Figures
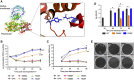
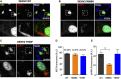
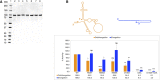
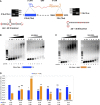
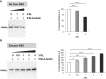
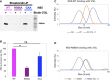
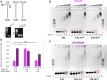
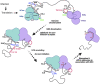
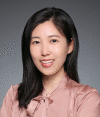
Similar articles
-
Interactions between the Dengue Virus Polymerase NS5 and Stem-Loop A.J Virol. 2017 May 12;91(11):e00047-17. doi: 10.1128/JVI.00047-17. Print 2017 Jun 1. J Virol. 2017. PMID: 28356528 Free PMC article.
-
The F1 motif of dengue virus polymerase NS5 is involved in promoter-dependent RNA synthesis.J Virol. 2011 Jun;85(12):5745-56. doi: 10.1128/JVI.02343-10. Epub 2011 Apr 6. J Virol. 2011. PMID: 21471248 Free PMC article.
-
The C-terminal 18 Amino Acid Region of Dengue Virus NS5 Regulates its Subcellular Localization and Contains a Conserved Arginine Residue Essential for Infectious Virus Production.PLoS Pathog. 2016 Sep 13;12(9):e1005886. doi: 10.1371/journal.ppat.1005886. eCollection 2016 Sep. PLoS Pathog. 2016. PMID: 27622521 Free PMC article.
-
Functional RNA elements in the dengue virus genome.Viruses. 2011 Sep;3(9):1739-56. doi: 10.3390/v3091739. Epub 2011 Sep 15. Viruses. 2011. PMID: 21994804 Free PMC article. Review.
-
The Transactions of NS3 and NS5 in Flaviviral RNA Replication.Adv Exp Med Biol. 2018;1062:147-163. doi: 10.1007/978-981-10-8727-1_11. Adv Exp Med Biol. 2018. PMID: 29845531 Review.
Cited by
-
Investigating RNA-RNA interactions through computational and biophysical analysis.Nucleic Acids Res. 2023 May 22;51(9):4588-4601. doi: 10.1093/nar/gkad223. Nucleic Acids Res. 2023. PMID: 36999609 Free PMC article.
-
The Flavivirus Non-Structural Protein 5 (NS5): Structure, Functions, and Targeting for Development of Vaccines and Therapeutics.Vaccines (Basel). 2024 Aug 1;12(8):865. doi: 10.3390/vaccines12080865. Vaccines (Basel). 2024. PMID: 39203991 Free PMC article. Review.
-
Conserved RNA secondary structure in Cherry virus A 5'-UTR associated with translation regulation.Virol J. 2022 May 26;19(1):91. doi: 10.1186/s12985-022-01824-z. Virol J. 2022. PMID: 35619168 Free PMC article.
-
Serotype-Specific Regulation of Dengue Virus NS5 Protein Subcellular Localization.ACS Infect Dis. 2024 Jun 14;10(6):2047-2062. doi: 10.1021/acsinfecdis.4c00054. Epub 2024 May 29. ACS Infect Dis. 2024. PMID: 38811007 Free PMC article.
-
The myriad roles of RNA structure in the flavivirus life cycle.RNA Biol. 2024 Jan;21(1):14-30. doi: 10.1080/15476286.2024.2357857. Epub 2024 May 26. RNA Biol. 2024. PMID: 38797925 Free PMC article. Review.
References
Publication types
MeSH terms
Substances
LinkOut - more resources
Full Text Sources
Research Materials