Quantifying force transmission through fibroblasts: changes of traction forces under external shearing
- PMID: 34713316
- PMCID: PMC8964583
- DOI: 10.1007/s00249-021-01576-8
Quantifying force transmission through fibroblasts: changes of traction forces under external shearing
Abstract
Mammalian cells have evolved complex mechanical connections to their microenvironment, including focal adhesion clusters that physically connect the cytoskeleton and the extracellular matrix. This mechanical link is also part of the cellular machinery to transduce, sense and respond to external forces. Although methods to measure cell attachment and cellular traction forces are well established, these are not capable of quantifying force transmission through the cell body to adhesion sites. We here present a novel approach to quantify intracellular force transmission by combining microneedle shearing at the apical cell surface with traction force microscopy at the basal cell surface. The change of traction forces exerted by fibroblasts to underlying polyacrylamide substrates as a response to a known shear force exerted with a calibrated microneedle reveals that cells redistribute forces dynamically under external shearing and during sequential rupture of their adhesion sites. Our quantitative results demonstrate a transition from dipolar to monopolar traction patterns, an inhomogeneous distribution of the external shear force to the adhesion sites as well as dynamical changes in force loading prior to and after the rupture of single adhesion sites. Our strategy of combining traction force microscopy with external force application opens new perspectives for future studies of force transmission and mechanotransduction in cells.
Keywords: Cell adhesion; Mechanobiology; Micromanipulation; Traction force microscopy.
© 2021. The Author(s).
Figures
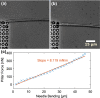
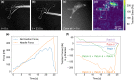
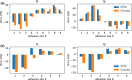
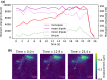
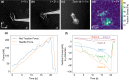
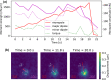
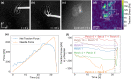
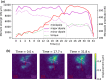
Similar articles
-
Traction force microscopy for understanding cellular mechanotransduction.BMB Rep. 2020 Feb;53(2):74-81. doi: 10.5483/BMBRep.2020.53.2.308. BMB Rep. 2020. PMID: 31964473 Free PMC article. Review.
-
4D Force Detection of Cell Adhesion and Contractility.Nano Lett. 2023 Apr 12;23(7):2467-2475. doi: 10.1021/acs.nanolett.2c03733. Epub 2023 Mar 28. Nano Lett. 2023. PMID: 36975035 Free PMC article.
-
Measuring (biological) materials mechanics with atomic force microscopy. 5. Traction force microscopy (cell traction forces).Microsc Res Tech. 2023 Sep;86(9):1069-1078. doi: 10.1002/jemt.24368. Epub 2023 Jun 22. Microsc Res Tech. 2023. PMID: 37345422 Free PMC article. Review.
-
Force mapping during the formation and maturation of cell adhesion sites with multiple optical tweezers.PLoS One. 2013;8(1):e54850. doi: 10.1371/journal.pone.0054850. Epub 2013 Jan 25. PLoS One. 2013. PMID: 23372781 Free PMC article.
-
Quantifying Immune Cell Force Generation Using Traction Force Microscopy.Methods Mol Biol. 2023;2654:363-373. doi: 10.1007/978-1-0716-3135-5_23. Methods Mol Biol. 2023. PMID: 37106194
Cited by
-
Nanoengineering for Mechanobiology "N4M-20".Eur Biophys J. 2022 Mar;51(2):97-98. doi: 10.1007/s00249-022-01596-y. Eur Biophys J. 2022. PMID: 35316358 No abstract available.
References
MeSH terms
Grants and funding
LinkOut - more resources
Full Text Sources