Human ALS/FTD brain organoid slice cultures display distinct early astrocyte and targetable neuronal pathology
- PMID: 34675437
- PMCID: PMC8553627
- DOI: 10.1038/s41593-021-00923-4
Human ALS/FTD brain organoid slice cultures display distinct early astrocyte and targetable neuronal pathology
Abstract
Amyotrophic lateral sclerosis overlapping with frontotemporal dementia (ALS/FTD) is a fatal and currently untreatable disease characterized by rapid cognitive decline and paralysis. Elucidating initial cellular pathologies is central to therapeutic target development, but obtaining samples from presymptomatic patients is not feasible. Here, we report the development of a cerebral organoid slice model derived from human induced pluripotent stem cells (iPSCs) that recapitulates mature cortical architecture and displays early molecular pathology of C9ORF72 ALS/FTD. Using a combination of single-cell RNA sequencing and biological assays, we reveal distinct transcriptional, proteostasis and DNA repair disturbances in astroglia and neurons. We show that astroglia display increased levels of the autophagy signaling protein P62 and that deep layer neurons accumulate dipeptide repeat protein poly(GA), DNA damage and undergo nuclear pyknosis that could be pharmacologically rescued by GSK2606414. Thus, patient-specific iPSC-derived cortical organoid slice cultures are a reproducible translational platform to investigate preclinical ALS/FTD mechanisms as well as novel therapeutic approaches.
© 2021. The Author(s).
Conflict of interest statement
G.B. is a co-founder of and consultant for Adrestia Therapeutics Ltd. The remaining authors declare no competing interests.
Figures
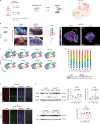
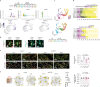
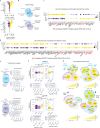
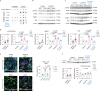
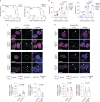
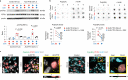
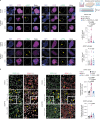
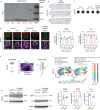
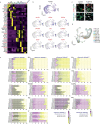
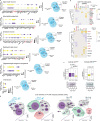
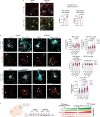
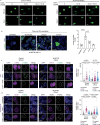
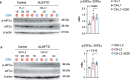
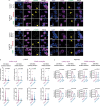
Similar articles
-
Molecular pathology, developmental changes and synaptic dysfunction in (pre-) symptomatic human C9ORF72-ALS/FTD cerebral organoids.Acta Neuropathol Commun. 2024 Sep 18;12(1):152. doi: 10.1186/s40478-024-01857-1. Acta Neuropathol Commun. 2024. PMID: 39289761 Free PMC article.
-
Neural Differentiation and spinal cord organoid generation from induced pluripotent stem cells (iPSCs) for ALS modelling and inflammatory screening.Mol Neurobiol. 2024 Jul;61(7):4732-4749. doi: 10.1007/s12035-023-03836-4. Epub 2023 Dec 21. Mol Neurobiol. 2024. PMID: 38127186
-
Toxic gain-of-function mechanisms in C9orf72 ALS-FTD neurons drive autophagy and lysosome dysfunction.Autophagy. 2024 Sep;20(9):2102-2104. doi: 10.1080/15548627.2024.2340415. Epub 2024 Apr 18. Autophagy. 2024. PMID: 38615685 Free PMC article.
-
Proteostasis deregulation as a driver of C9ORF72 pathogenesis.J Neurochem. 2021 Dec;159(6):941-957. doi: 10.1111/jnc.15529. Epub 2021 Nov 9. J Neurochem. 2021. PMID: 34679204 Review.
-
C9orf72 ALS-FTD: recent evidence for dysregulation of the autophagy-lysosome pathway at multiple levels.Autophagy. 2021 Nov;17(11):3306-3322. doi: 10.1080/15548627.2021.1872189. Epub 2021 Feb 26. Autophagy. 2021. PMID: 33632058 Free PMC article. Review.
Cited by
-
Endogenous retroviruses are dysregulated in ALS.iScience. 2024 May 28;27(7):110147. doi: 10.1016/j.isci.2024.110147. eCollection 2024 Jul 19. iScience. 2024. PMID: 38989463 Free PMC article.
-
Landscape of human organoids: Ideal model in clinics and research.Innovation (Camb). 2024 Apr 1;5(3):100620. doi: 10.1016/j.xinn.2024.100620. eCollection 2024 May 6. Innovation (Camb). 2024. PMID: 38706954 Free PMC article. Review.
-
Advances and Applications of Brain Organoids.Neurosci Bull. 2023 Nov;39(11):1703-1716. doi: 10.1007/s12264-023-01065-2. Epub 2023 May 24. Neurosci Bull. 2023. PMID: 37222855 Free PMC article. Review.
-
Building consensus on the application of organoid-based drug sensitivity testing in cancer precision medicine and drug development.Theranostics. 2024 May 27;14(8):3300-3316. doi: 10.7150/thno.96027. eCollection 2024. Theranostics. 2024. PMID: 38855182 Free PMC article. Review.
-
Brain organoids: A revolutionary tool for modeling neurological disorders and development of therapeutics.Biotechnol Bioeng. 2024 Feb;121(2):489-506. doi: 10.1002/bit.28606. Epub 2023 Nov 28. Biotechnol Bioeng. 2024. PMID: 38013504 Free PMC article. Review.
References
Publication types
MeSH terms
Substances
Grants and funding
LinkOut - more resources
Full Text Sources
Other Literature Sources
Medical
Molecular Biology Databases
Miscellaneous