The Relationship of Glutathione- S-Transferase and Multi-Drug Resistance-Related Protein 1 in Nitric Oxide (NO) Transport and Storage
- PMID: 34641326
- PMCID: PMC8510172
- DOI: 10.3390/molecules26195784
The Relationship of Glutathione- S-Transferase and Multi-Drug Resistance-Related Protein 1 in Nitric Oxide (NO) Transport and Storage
Abstract
Nitric oxide is a diatomic gas that has traditionally been viewed, particularly in the context of chemical fields, as a toxic, pungent gas that is the product of ammonia oxidation. However, nitric oxide has been associated with many biological roles including cell signaling, macrophage cytotoxicity, and vasodilation. More recently, a model for nitric oxide trafficking has been proposed where nitric oxide is regulated in the form of dinitrosyl-dithiol-iron-complexes, which are much less toxic and have a significantly greater half-life than free nitric oxide. Our laboratory has previously examined this hypothesis in tumor cells and has demonstrated that dinitrosyl-dithiol-iron-complexes are transported and stored by multi-drug resistance-related protein 1 and glutathione-S-transferase P1. A crystal structure of a dinitrosyl-dithiol-iron complex with glutathione-S-transferase P1 has been solved that demonstrates that a tyrosine residue in glutathione-S-transferase P1 is responsible for binding dinitrosyl-dithiol-iron-complexes. Considering the roles of nitric oxide in vasodilation and many other processes, a physiological model of nitric oxide transport and storage would be valuable in understanding nitric oxide physiology and pathophysiology.
Keywords: dinitrosyl–dithiol iron complexes; free radicals; glutathione-S-transferase; multi-drug resistance related protein 1; nitric oxide; nitric oxide synthase; nitrogen monoxide; protein metal ion interactions; vasodilation.
Conflict of interest statement
The authors declare no conflict of interest.
Figures
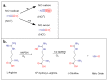
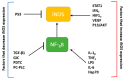
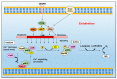
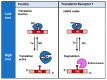
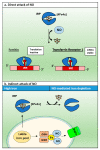
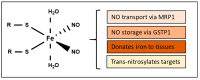
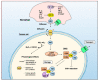
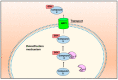
Similar articles
-
Nitric oxide storage and transport in cells are mediated by glutathione S-transferase P1-1 and multidrug resistance protein 1 via dinitrosyl iron complexes.J Biol Chem. 2012 Jan 2;287(1):607-618. doi: 10.1074/jbc.M111.310987. Epub 2011 Nov 14. J Biol Chem. 2012. PMID: 22084240 Free PMC article.
-
Nitrogen monoxide (NO) storage and transport by dinitrosyl-dithiol-iron complexes: long-lived NO that is trafficked by interacting proteins.J Biol Chem. 2012 Mar 2;287(10):6960-8. doi: 10.1074/jbc.R111.329847. Epub 2012 Jan 19. J Biol Chem. 2012. PMID: 22262835 Free PMC article.
-
Regulation and control of nitric oxide (NO) in macrophages: Protecting the "professional killer cell" from its own cytotoxic arsenal via MRP1 and GSTP1.Biochim Biophys Acta Gen Subj. 2017 May;1861(5 Pt A):995-999. doi: 10.1016/j.bbagen.2017.02.021. Epub 2017 Feb 17. Biochim Biophys Acta Gen Subj. 2017. PMID: 28219722 Review.
-
A Nitric Oxide Storage and Transport System That Protects Activated Macrophages from Endogenous Nitric Oxide Cytotoxicity.J Biol Chem. 2016 Dec 30;291(53):27042-27061. doi: 10.1074/jbc.M116.763714. Epub 2016 Nov 19. J Biol Chem. 2016. PMID: 27866158 Free PMC article.
-
Glutathione S-transferase and MRP1 form an integrated system involved in the storage and transport of dinitrosyl-dithiolato iron complexes in cells.Free Radic Biol Med. 2014 Oct;75:14-29. doi: 10.1016/j.freeradbiomed.2014.07.002. Epub 2014 Jul 15. Free Radic Biol Med. 2014. PMID: 25035074 Review.
Cited by
-
S-Denitrosylation: A Crosstalk between Glutathione and Redoxin Systems.Antioxidants (Basel). 2022 Sep 28;11(10):1921. doi: 10.3390/antiox11101921. Antioxidants (Basel). 2022. PMID: 36290644 Free PMC article. Review.
-
Glutathione transferase P1 is modified by palmitate.PLoS One. 2024 Sep 13;19(9):e0308500. doi: 10.1371/journal.pone.0308500. eCollection 2024. PLoS One. 2024. PMID: 39269939 Free PMC article.
-
SPAG6 overexpression decreases the pro-apoptotic effect of daunorubicin in acute myeloid leukemia cells through the ROS/JNK MAPK axis in a GSTP1-dependent manner.Front Pharmacol. 2024 Oct 23;15:1390456. doi: 10.3389/fphar.2024.1390456. eCollection 2024. Front Pharmacol. 2024. PMID: 39508041 Free PMC article.
References
Publication types
MeSH terms
Substances
Grants and funding
LinkOut - more resources
Full Text Sources
Research Materials
Miscellaneous