Tissue clearing and 3D imaging in developmental biology
- PMID: 34596666
- PMCID: PMC8497915
- DOI: 10.1242/dev.199369
Tissue clearing and 3D imaging in developmental biology
Abstract
Tissue clearing increases the transparency of late developmental stages and enables deep imaging in fixed organisms. Successful implementation of these methodologies requires a good grasp of sample processing, imaging and the possibilities offered by image analysis. In this Primer, we highlight how tissue clearing can revolutionize the histological analysis of developmental processes and we advise on how to implement effective clearing protocols, imaging strategies and analysis methods for developmental biology.
Keywords: 3D imaging; Light-sheet microscopy; Tissue clearing.
© 2021. Published by The Company of Biologists Ltd.
Conflict of interest statement
Competing interests The authors declare no competing or financial interests.
Figures
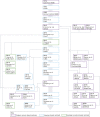
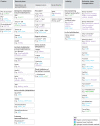
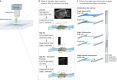
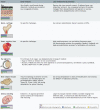
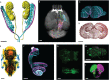
Similar articles
-
Successful 3D imaging of cleared biological samples with light sheet fluorescence microscopy.J Cell Biol. 2023 Nov 6;222(11):e202307143. doi: 10.1083/jcb.202307143. Epub 2023 Oct 17. J Cell Biol. 2023. PMID: 37847528 Free PMC article. Review.
-
Whole-Brain Single-Cell Imaging and Analysis of Intact Neonatal Mouse Brains Using MRI, Tissue Clearing, and Light-Sheet Microscopy.J Vis Exp. 2022 Aug 1;(186):10.3791/64096. doi: 10.3791/64096. J Vis Exp. 2022. PMID: 35969091 Free PMC article.
-
A survey of clearing techniques for 3D imaging of tissues with special reference to connective tissue.Prog Histochem Cytochem. 2016 Aug;51(2):9-23. doi: 10.1016/j.proghi.2016.04.001. Epub 2016 Apr 14. Prog Histochem Cytochem. 2016. PMID: 27142295 Review.
-
Imaging the mammary gland and mammary tumours in 3D: optical tissue clearing and immunofluorescence methods.Breast Cancer Res. 2016 Dec 13;18(1):127. doi: 10.1186/s13058-016-0754-9. Breast Cancer Res. 2016. PMID: 27964754 Free PMC article.
-
Selective plane illumination microscopy techniques in developmental biology.Development. 2009 Jun;136(12):1963-75. doi: 10.1242/dev.022426. Development. 2009. PMID: 19465594 Free PMC article. Review.
Cited by
-
Successful 3D imaging of cleared biological samples with light sheet fluorescence microscopy.J Cell Biol. 2023 Nov 6;222(11):e202307143. doi: 10.1083/jcb.202307143. Epub 2023 Oct 17. J Cell Biol. 2023. PMID: 37847528 Free PMC article. Review.
-
Glutaraldehyde-enhanced autofluorescence as a general tool for 3D morphological imaging.Biol Open. 2024 Nov 15;13(11):bio060428. doi: 10.1242/bio.060428. Epub 2024 Nov 11. Biol Open. 2024. PMID: 39428988 Free PMC article.
-
A Guide to Examining Intramuscular Fat Formation and its Cellular Origin in Skeletal Muscle.J Vis Exp. 2022 May 26;(183):10.3791/63996. doi: 10.3791/63996. J Vis Exp. 2022. PMID: 35695517 Free PMC article.
-
Flip-Flap: A Simple Dual-View Imaging Method for 3D Reconstruction of Thick Plant Samples.Plants (Basel). 2022 Feb 13;11(4):506. doi: 10.3390/plants11040506. Plants (Basel). 2022. PMID: 35214839 Free PMC article.
-
Visualizing Cathepsin K-Cre Expression at the Single-Cell Level with GFP Reporters.JBMR Plus. 2022 Dec 21;7(1):e10706. doi: 10.1002/jbm4.10706. eCollection 2023 Jan. JBMR Plus. 2022. PMID: 36699636 Free PMC article.
References
-
- Ariel, P. (2018). UltraMicroscope II – A User Guide [Internet]. Chapel Hill, NC: University of North Carolina at Chapel Hill. 10.17615/C69M15 - PubMed
Publication types
MeSH terms
LinkOut - more resources
Full Text Sources