Low-Dose Radiotherapy Reverses Tumor Immune Desertification and Resistance to Immunotherapy
- PMID: 34479871
- PMCID: PMC9401506
- DOI: 10.1158/2159-8290.CD-21-0003
Low-Dose Radiotherapy Reverses Tumor Immune Desertification and Resistance to Immunotherapy
Abstract
Developing strategies to inflame tumors is critical for increasing response to immunotherapy. Here, we report that low-dose radiotherapy (LDRT) of murine tumors promotes T-cell infiltration and enables responsiveness to combinatorial immunotherapy in an IFN-dependent manner. Treatment efficacy relied upon mobilizing both adaptive and innate immunity and depended on both cytotoxic CD4+ and CD8+ T cells. LDRT elicited predominantly CD4+ cells with features of exhausted effector cytotoxic cells, with a subset expressing NKG2D and exhibiting proliferative capacity, as well as a unique subset of activated dendritic cells expressing the NKG2D ligand RAE1. We translated these findings to a phase I clinical trial administering LDRT, low-dose cyclophosphamide, and immune checkpoint blockade to patients with immune-desert tumors. In responsive patients, the combinatorial treatment triggered T-cell infiltration, predominantly of CD4+ cells with Th1 signatures. Our data support the rational combination of LDRT with immunotherapy for effectively treating low T cell-infiltrated tumors. SIGNIFICANCE: Low-dose radiation reprogrammed the tumor microenvironment of tumors with scarce immune infiltration and together with immunotherapy induced simultaneous mobilization of innate and adaptive immunity, predominantly CD4+ effector T cells, to achieve tumor control dependent on NKG2D. The combination induced important responses in patients with metastatic immune-cold tumors.This article is highlighted in the In This Issue feature, p. 1.
©2021 The Authors; Published by the American Association for Cancer Research.
Figures
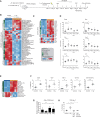
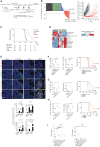
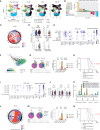
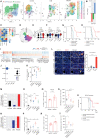
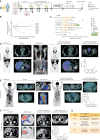
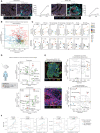
Comment in
- Cancer Discov. 12:1.
- Cancer Discov. 12:1.
Similar articles
-
CDK4/6 inhibition promotes immune infiltration in ovarian cancer and synergizes with PD-1 blockade in a B cell-dependent manner.Theranostics. 2020 Aug 25;10(23):10619-10633. doi: 10.7150/thno.44871. eCollection 2020. Theranostics. 2020. PMID: 32929370 Free PMC article.
-
CD4+ T-cell epitope-based heterologous prime-boost vaccination potentiates anti-tumor immunity and PD-1/PD-L1 immunotherapy.J Immunother Cancer. 2022 May;10(5):e004022. doi: 10.1136/jitc-2021-004022. J Immunother Cancer. 2022. PMID: 35580929 Free PMC article.
-
Checkpoint blockade immunotherapy enhances the frequency and effector function of murine tumor-infiltrating T cells but does not alter TCRβ diversity.Cancer Immunol Immunother. 2019 Jul;68(7):1095-1106. doi: 10.1007/s00262-019-02346-4. Epub 2019 May 18. Cancer Immunol Immunother. 2019. PMID: 31104075 Free PMC article.
-
CD8+ cytotoxic T lymphocytes in cancer immunotherapy: A review.J Cell Physiol. 2019 Jun;234(6):8509-8521. doi: 10.1002/jcp.27782. Epub 2018 Nov 22. J Cell Physiol. 2019. PMID: 30520029 Review.
-
Lighting up the tumor fire with low-dose irradiation.Trends Immunol. 2022 Mar;43(3):173-179. doi: 10.1016/j.it.2022.01.006. Epub 2022 Jan 31. Trends Immunol. 2022. PMID: 35105519 Review.
Cited by
-
Metabolic Reprogramming of Cancer-Associated Fibroblast in the Tumor Microenvironment: From Basics to Clinic.Clin Med Insights Oncol. 2024 Oct 21;18:11795549241287058. doi: 10.1177/11795549241287058. eCollection 2024. Clin Med Insights Oncol. 2024. PMID: 39450056 Free PMC article. Review.
-
Non-homogenous intratumor ionizing radiation doses synergize with PD1 and CXCR2 blockade.Nat Commun. 2024 Oct 14;15(1):8845. doi: 10.1038/s41467-024-53015-9. Nat Commun. 2024. PMID: 39397001 Free PMC article.
-
Immunotherapy for ovarian cancer: towards a tailored immunophenotype-based approach.Nat Rev Clin Oncol. 2024 Nov;21(11):801-817. doi: 10.1038/s41571-024-00937-4. Epub 2024 Sep 4. Nat Rev Clin Oncol. 2024. PMID: 39232212 Review.
-
Abscopal effect: from a rare phenomenon to a new frontier in cancer therapy.Biomark Res. 2024 Sep 4;12(1):98. doi: 10.1186/s40364-024-00628-3. Biomark Res. 2024. PMID: 39228005 Free PMC article. Review.
-
Locoregional therapies combined with immune checkpoint inhibitors for liver metastases.Cancer Cell Int. 2024 Aug 31;24(1):302. doi: 10.1186/s12935-024-03484-1. Cancer Cell Int. 2024. PMID: 39217341 Free PMC article. Review.
References
-
- Herrera FG, Bourhis J, Coukos G. Radiotherapy combination opportunities leveraging immunity for the next oncology practice. CA Cancer J Clin 2017;67:65–85. - PubMed
Publication types
MeSH terms
LinkOut - more resources
Full Text Sources
Medical
Molecular Biology Databases
Research Materials