How Epstein-Barr Virus and Kaposi's Sarcoma-Associated Herpesvirus Are Maintained Together to Transform the Same B-Cell
- PMID: 34452344
- PMCID: PMC8402831
- DOI: 10.3390/v13081478
How Epstein-Barr Virus and Kaposi's Sarcoma-Associated Herpesvirus Are Maintained Together to Transform the Same B-Cell
Abstract
Epstein-Barr virus (EBV) and Kaposi's sarcoma-associated herpesvirus (KSHV) independently cause human cancers, and both are maintained as plasmids in tumor cells. They differ, however, in their mechanisms of segregation; EBV partitions its genomes quasi-faithfully, while KSHV often clusters its genomes and partitions them randomly. Both viruses can infect the same B-cell to transform it in vitro and to cause primary effusion lymphomas (PELs) in vivo. We have developed simulations based on our measurements of these replicons in B-cells transformed in vitro to elucidate the synthesis and partitioning of these two viral genomes when in the same cell. These simulations successfully capture the biology of EBV and KSHV in PELs. They have revealed that EBV and KSHV replicate and partition independently, that they both contribute selective advantages to their host cell, and that KSHV pays a penalty to cluster its genomes.
Keywords: EBV; KSHV; dual-infection; model.
Conflict of interest statement
The authors declare no conflict of interest.
Figures
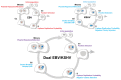
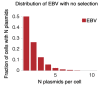
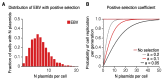
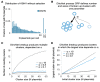
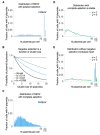
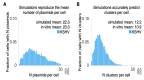
Similar articles
-
Persistent KSHV Infection Increases EBV-Associated Tumor Formation In Vivo via Enhanced EBV Lytic Gene Expression.Cell Host Microbe. 2017 Jul 12;22(1):61-73.e7. doi: 10.1016/j.chom.2017.06.009. Cell Host Microbe. 2017. PMID: 28704654
-
Mutual inhibition between Kaposi's sarcoma-associated herpesvirus and Epstein-Barr virus lytic replication initiators in dually-infected primary effusion lymphoma.PLoS One. 2008 Feb 6;3(2):e1569. doi: 10.1371/journal.pone.0001569. PLoS One. 2008. PMID: 18253508 Free PMC article.
-
Epstein-Barr virus recombinants from BC-1 and BC-2 can immortalize human primary B lymphocytes with different levels of efficiency and in the absence of coinfection by Kaposi's sarcoma-associated herpesvirus.J Virol. 2000 Jan;74(2):735-43. doi: 10.1128/jvi.74.2.735-743.2000. J Virol. 2000. PMID: 10623735 Free PMC article.
-
[EBV/KSHV co-infection: an effective partnership].Med Sci (Paris). 2018 Jan;34(1):79-82. doi: 10.1051/medsci/20183401017. Epub 2018 Jan 31. Med Sci (Paris). 2018. PMID: 29384100 Review. French.
-
Manipulation of the host cell membrane by human γ-herpesviruses EBV and KSHV for pathogenesis.Virol Sin. 2016 Oct;31(5):395-405. doi: 10.1007/s12250-016-3817-2. Epub 2016 Sep 12. Virol Sin. 2016. PMID: 27624182 Free PMC article. Review.
Cited by
-
Carcinogenic mechanisms of virus-associated lymphoma.Front Immunol. 2024 Feb 28;15:1361009. doi: 10.3389/fimmu.2024.1361009. eCollection 2024. Front Immunol. 2024. PMID: 38482011 Free PMC article. Review.
-
Co-Infection of the Epstein-Barr Virus and the Kaposi Sarcoma-Associated Herpesvirus.Viruses. 2022 Dec 2;14(12):2709. doi: 10.3390/v14122709. Viruses. 2022. PMID: 36560713 Free PMC article. Review.
References
Publication types
MeSH terms
Grants and funding
LinkOut - more resources
Full Text Sources