Allele-specific mitochondrial stress induced by Multiple Mitochondrial Dysfunctions Syndrome 1 pathogenic mutations modeled in Caenorhabditis elegans
- PMID: 34449775
- PMCID: PMC8428684
- DOI: 10.1371/journal.pgen.1009771
Allele-specific mitochondrial stress induced by Multiple Mitochondrial Dysfunctions Syndrome 1 pathogenic mutations modeled in Caenorhabditis elegans
Abstract
Multiple Mitochondrial Dysfunctions Syndrome 1 (MMDS1) is a rare, autosomal recessive disorder caused by mutations in the NFU1 gene. NFU1 is responsible for delivery of iron-sulfur clusters (ISCs) to recipient proteins which require these metallic cofactors for their function. Pathogenic variants of NFU1 lead to dysfunction of its target proteins within mitochondria. To date, 20 NFU1 variants have been reported and the unique contributions of each variant to MMDS1 pathogenesis is unknown. Given that over half of MMDS1 individuals are compound heterozygous for different NFU1 variants, it is valuable to investigate individual variants in an isogenic background. In order to understand the shared and unique phenotypes of NFU1 variants, we used CRISPR/Cas9 gene editing to recreate exact patient variants of NFU1 in the orthologous gene, nfu-1 (formerly lpd-8), in C. elegans. Five mutant C. elegans alleles focused on the presumptive iron-sulfur cluster interaction domain were generated and analyzed for mitochondrial phenotypes including respiratory dysfunction and oxidative stress. Phenotypes were variable between the mutant nfu-1 alleles and generally presented as an allelic series indicating that not all variants have lost complete function. Furthermore, reactive iron within mitochondria was evident in some, but not all, nfu-1 mutants indicating that iron dyshomeostasis may contribute to disease pathogenesis in some MMDS1 individuals.
Conflict of interest statement
The authors have declared that no competing interests exist.
Figures

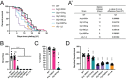
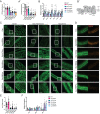
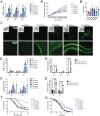
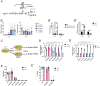
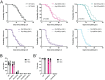
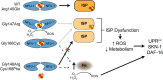
Similar articles
-
Patient-specific variants of NFU1/NFU-1 disrupt cholinergic signaling in a model of multiple mitochondrial dysfunctions syndrome 1.Dis Model Mech. 2023 Feb 1;16(2):dmm049594. doi: 10.1242/dmm.049594. Epub 2023 Feb 1. Dis Model Mech. 2023. PMID: 36645076 Free PMC article.
-
Assembly of the [4Fe-4S] cluster of NFU1 requires the coordinated donation of two [2Fe-2S] clusters from the scaffold proteins, ISCU2 and ISCA1.Hum Mol Genet. 2020 Nov 25;29(19):3165-3182. doi: 10.1093/hmg/ddaa172. Hum Mol Genet. 2020. PMID: 32776106 Free PMC article.
-
Analysis of NFU-1 metallocofactor binding-site substitutions-impacts on iron-sulfur cluster coordination and protein structure and function.FEBS J. 2017 Nov;284(22):3817-3837. doi: 10.1111/febs.14270. Epub 2017 Oct 16. FEBS J. 2017. PMID: 28906593
-
Mitochondrial iron-sulfur protein biogenesis and human disease.Biochimie. 2014 May;100:61-77. doi: 10.1016/j.biochi.2014.01.010. Epub 2014 Jan 23. Biochimie. 2014. PMID: 24462711 Review.
-
Novel IBA57 mutations in two chinese patients and literature review of multiple mitochondrial dysfunction syndrome.Metab Brain Dis. 2022 Feb;37(2):311-317. doi: 10.1007/s11011-021-00856-8. Epub 2021 Oct 28. Metab Brain Dis. 2022. PMID: 34709542 Review.
Cited by
-
BOLA3 and NFU1 link mitoribosome iron-sulfur cluster assembly to multiple mitochondrial dysfunctions syndrome.Nucleic Acids Res. 2023 Nov 27;51(21):11797-11812. doi: 10.1093/nar/gkad842. Nucleic Acids Res. 2023. PMID: 37823603 Free PMC article.
-
Patient-specific variants of NFU1/NFU-1 disrupt cholinergic signaling in a model of multiple mitochondrial dysfunctions syndrome 1.Dis Model Mech. 2023 Feb 1;16(2):dmm049594. doi: 10.1242/dmm.049594. Epub 2023 Feb 1. Dis Model Mech. 2023. PMID: 36645076 Free PMC article.
-
Exploring therapeutic potential of mitophagy modulators using Drosophila models of Parkinson's disease.Front Aging Neurosci. 2022 Oct 19;14:986849. doi: 10.3389/fnagi.2022.986849. eCollection 2022. Front Aging Neurosci. 2022. PMID: 36337696 Free PMC article. Review.
-
TUNEL Labeling to Detect Double-stranded DNA Breaks in Caenorhabditis elegans Gonads.Bio Protoc. 2022 Mar 20;12(6):e4351. doi: 10.21769/BioProtoc.4351. eCollection 2022 Mar 20. Bio Protoc. 2022. PMID: 35434193 Free PMC article.
References
-
- Cai K, Liu G, Frederick RO, Xiao R, Montelione GT, Markley JL. Structural/Functional Properties of Human NFU1, an Intermediate [4Fe-4S] Carrier in Human Mitochondrial Iron-Sulfur Cluster Biogenesis. Structure (London, England: 1993). 2016;24: 2080–2091. doi: 10.1016/j.str.2016.08.020 - DOI - PMC - PubMed
Publication types
MeSH terms
Substances
Supplementary concepts
Grants and funding
LinkOut - more resources
Full Text Sources
Other Literature Sources
Medical
Research Materials