EPR Study of KO2 as a Source of Superoxide and •BMPO-OH/OOH Radical That Cleaves Plasmid DNA and Detects Radical Interaction with H2S and Se-Derivatives
- PMID: 34439533
- PMCID: PMC8389328
- DOI: 10.3390/antiox10081286
EPR Study of KO2 as a Source of Superoxide and •BMPO-OH/OOH Radical That Cleaves Plasmid DNA and Detects Radical Interaction with H2S and Se-Derivatives
Abstract
Superoxide radical anion (O2•-) and its derivatives regulate numerous physiological and pathological processes, which are extensively studied. The aim of our work was to utilize KO2 as a source of O2•- and the electron paramagnetic resonance (EPR) spin trapping 5-tert-butoxycarbonyl-5-methyl-1-pyrroline N-oxide (BMPO) technique for the preparation of •BMPO-OOH and/or •BMPO-OH radicals in water solution without DMSO. The method distinguishes the interactions of various compounds with •BMPO-OOH and/or •BMPO-OH radicals over time. Here, we show that the addition of a buffered BMPO-HCl mixture to powdered KO2 formed relatively stable •BMPO-OOH and •BMPO-OH radicals and H2O2, where the •BMPO-OOH/OH ratio depended on the pH. At a final pH of ~6.5-8.0, the concentration of •BMPO-OOH radicals was ≥20 times higher than that of •BMPO-OH, whereas at pH 9.0-10.0, the •BMPO-OH radicals prevailed. The •BMPO-OOH/OH radicals effectively cleaved the plasmid DNA. H2S decreased the concentration of •BMPO-OOH/OH radicals, whereas the selenium derivatives 1-methyl-4-(3-(phenylselanyl) propyl) piperazine and 1-methyl-4-(4-(phenylselanyl) butyl) piperazine increased the proportion of •BMPO-OH over the •BMPO-OOH radicals. In conclusion, the presented approach of using KO2 as a source of O2•-/H2O2 and EPR spin trap BMPO for the preparation of •BMPO-OOH/OH radicals in a physiological solution could be useful to study the biological effects of radicals and their interactions with compounds.
Keywords: EPR spectra simulation; KO2; antioxidants; cleavage DNA; hydrogen sulfide; radical; selenium-derivatives; superoxide; •BMPO-OOH spin adduct.
Conflict of interest statement
The authors declare no conflict of interest.
Figures
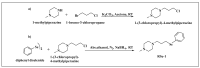
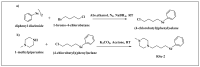
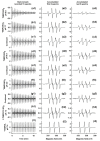
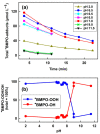
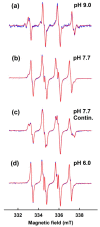
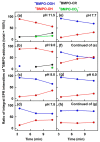
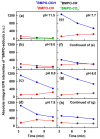
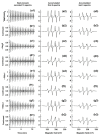
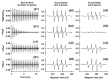
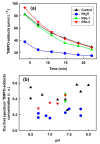
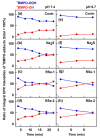
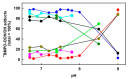

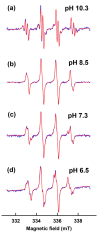
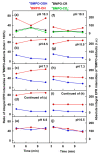
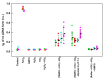
Similar articles
-
•BMPO-OOH Spin-Adduct as a Model for Study of Decomposition of Organic Hydroperoxides and the Effects of Sulfide/Selenite Derivatives. An EPR Spin-Trapping Approach.Antioxidants (Basel). 2020 Sep 26;9(10):918. doi: 10.3390/antiox9100918. Antioxidants (Basel). 2020. PMID: 32993108 Free PMC article.
-
Polysulfides and products of H2S/S-nitrosoglutathione in comparison to H2S, glutathione and antioxidant Trolox are potent scavengers of superoxide anion radical and produce hydroxyl radical by decomposition of H2O2.Nitric Oxide. 2018 Jun 1;76:136-151. doi: 10.1016/j.niox.2017.09.006. Epub 2017 Sep 23. Nitric Oxide. 2018. PMID: 28951200
-
Photoenhanced Radical Formation in Aqueous Mixtures of Levoglucosan and Benzoquinone: Implications to Photochemical Aging of Biomass-Burning Organic Aerosols.J Phys Chem A. 2023 Jun 22;127(24):5209-5221. doi: 10.1021/acs.jpca.3c01794. Epub 2023 Jun 7. J Phys Chem A. 2023. PMID: 37285129 Free PMC article.
-
Use of rapid-scan EPR to improve detection sensitivity for spin-trapped radicals.Biophys J. 2013 Jul 16;105(2):338-42. doi: 10.1016/j.bpj.2013.06.005. Biophys J. 2013. PMID: 23870255 Free PMC article.
-
Detection and characterisation of radicals in biological materials using EPR methodology.Biochim Biophys Acta. 2014 Feb;1840(2):708-21. doi: 10.1016/j.bbagen.2013.03.034. Epub 2013 Apr 6. Biochim Biophys Acta. 2014. PMID: 23567797 Review.
References
-
- Carroll L., Pattison D.I., Davies J.B., Anderson R.F., Lopez-Alarcon C., Davies M.J. Superoxide radicals react with peptide-derived tryptophan radicals with very high rate constants to give hydroperoxides as major products. Free Radic. Biol. Med. 2018;118:126–136. doi: 10.1016/j.freeradbiomed.2018.02.033. - DOI - PubMed
-
- Zhao H., Joseph J., Zhang H., Karoui H., Kalyanaraman B. Synthesis and biochemical applications of a solid cyclic nitrone spin trap: A relatively superior trap for detecting superoxide anions and glutathiyl radicals. Free Radic. Biol. Med. 2001;31:599–606. doi: 10.1016/S0891-5849(01)00619-0. - DOI - PubMed
-
- Bézière N., Hardy M., Poulhès F., Karoui H., Tordo P., Ouari O., Frapart Y.-M., Rockenbauer A., Boucher J.-L., Mansuy D., et al. Metabolic stability of superoxide adducts derived from newly developed cyclic nitrone spin traps. Free Radic. Biol. Med. 2014;67:150–158. doi: 10.1016/j.freeradbiomed.2013.10.812. - DOI - PubMed
Grants and funding
LinkOut - more resources
Full Text Sources
Research Materials