Rapid 2H NMR Transverse Relaxation of Perdeuterated Lipid Acyl Chains of Membrane with Bound Viral Fusion Peptide Supports Large-Amplitude Motions of These Chains That Can Catalyze Membrane Fusion
- PMID: 34436856
- PMCID: PMC8577873
- DOI: 10.1021/acs.biochem.1c00316
Rapid 2H NMR Transverse Relaxation of Perdeuterated Lipid Acyl Chains of Membrane with Bound Viral Fusion Peptide Supports Large-Amplitude Motions of These Chains That Can Catalyze Membrane Fusion
Abstract
An early step in cellular infection by a membrane-enveloped virus like HIV or influenza is joining (fusion) of the viral and cell membranes. Fusion is catalyzed by a viral protein that typically includes an apolar "fusion peptide" (fp) segment that binds the target membrane prior to fusion. In this study, the effects of nonhomologous HIV and influenza fp's on lipid acyl chain motion are probed with 2H NMR transverse relaxation rates (R2's) of a perdeuterated DMPC membrane. Measurements were made between 35 and 0 °C, which brackets the membrane liquid-crystalline-to-gel phase transitions. Samples were made with either HIV "GPfp" at pH 7 or influenza "HAfp" at pH 5 or 7. GPfp induces vesicle fusion at pH 7, and HAfp induces more fusion at pH 5 vs 7. GPfp bound to DMPC adopts an intermolecular antiparallel β sheet structure, whereas HAfp is a monomer helical hairpin. The R2's of the no peptide and HAfp, pH 7, samples increase gradually as temperature is lowered. The R2's of GPfp and HAfp, pH 5, samples have very different temperature dependence, with a ∼10× increase in R2CD2 when temperature is reduced from 25 to 20 °C and smaller but still substantial R2's at 10 and 0 °C. The large R2's with GPfp and HAfp, pH 5, are consistent with large-amplitude motions of lipid acyl chains that can aid fusion catalysis by increasing the population of chains near the aqueous phase, which is the chain location for transition states between membrane fusion intermediates.
Figures
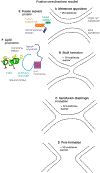
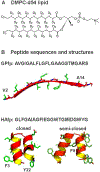
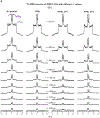
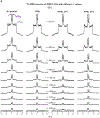
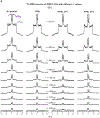
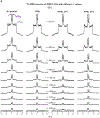
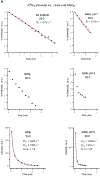
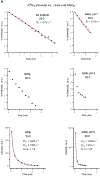
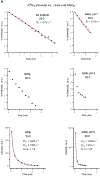
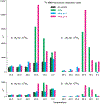
Similar articles
-
2H nuclear magnetic resonance spectroscopy supports larger amplitude fast motion and interference with lipid chain ordering for membrane that contains β sheet human immunodeficiency virus gp41 fusion peptide or helical hairpin influenza virus hemagglutinin fusion peptide at fusogenic pH.Biochim Biophys Acta Biomembr. 2020 Oct 1;1862(10):183404. doi: 10.1016/j.bbamem.2020.183404. Epub 2020 Jun 23. Biochim Biophys Acta Biomembr. 2020. PMID: 32585207 Free PMC article.
-
Solid-state nuclear magnetic resonance measurements of HIV fusion peptide 13CO to lipid 31P proximities support similar partially inserted membrane locations of the α helical and β sheet peptide structures.J Phys Chem A. 2013 Oct 3;117(39):9848-59. doi: 10.1021/jp312845w. Epub 2013 Feb 28. J Phys Chem A. 2013. PMID: 23418890 Free PMC article.
-
Comparative analysis of membrane-associated fusion peptide secondary structure and lipid mixing function of HIV gp41 constructs that model the early pre-hairpin intermediate and final hairpin conformations.J Mol Biol. 2010 Mar 19;397(1):301-15. doi: 10.1016/j.jmb.2010.01.018. Epub 2010 Jan 18. J Mol Biol. 2010. PMID: 20080102 Free PMC article.
-
Structural biology of the influenza virus fusion peptide.Acta Biochim Pol. 2014;61(3):421-6. Epub 2014 Sep 8. Acta Biochim Pol. 2014. PMID: 25195144 Review.
-
Are fusion peptides a good model to study viral cell fusion?Biochim Biophys Acta. 2003 Jul 11;1614(1):104-15. doi: 10.1016/s0005-2736(03)00168-8. Biochim Biophys Acta. 2003. PMID: 12873771 Review.
Cited by
-
Strategies for acquisition of resonance assignment spectra of highly dynamic membrane proteins: a GPCR case study.J Biomol NMR. 2023 Aug;77(4):191-202. doi: 10.1007/s10858-023-00421-8. Epub 2023 Jul 26. J Biomol NMR. 2023. PMID: 37493866 Free PMC article.
-
Lipid acyl chain protrusion induced by the influenza virus hemagglutinin fusion peptide detected by NMR paramagnetic relaxation enhancement.Biophys Chem. 2023 Aug;299:107028. doi: 10.1016/j.bpc.2023.107028. Epub 2023 May 13. Biophys Chem. 2023. PMID: 37247572 Free PMC article.
-
A large HIV gp41 construct with trimer-of-hairpins structure exhibits V2E mutation-dominant attenuation of vesicle fusion and helicity very similar to V2E attenuation of HIV fusion and infection and supports: (1) hairpin stabilization of membrane apposition with larger distance for V2E; and (2) V2E dominance by an antiparallel β sheet with interleaved fusion peptide strands from two gp41 trimers.Biophys Chem. 2023 Feb;293:106933. doi: 10.1016/j.bpc.2022.106933. Epub 2022 Nov 24. Biophys Chem. 2023. PMID: 36508984 Free PMC article.
-
Spectroscopic signatures of bilayer ordering in native biological membranes.Biochim Biophys Acta Biomembr. 2022 Jun 1;1864(6):183891. doi: 10.1016/j.bbamem.2022.183891. Epub 2022 Feb 22. Biochim Biophys Acta Biomembr. 2022. PMID: 35217001 Free PMC article.
-
Computational methods to study enveloped viral entry.Biochem Soc Trans. 2021 Dec 17;49(6):2527-2537. doi: 10.1042/BST20210190. Biochem Soc Trans. 2021. PMID: 34783344 Free PMC article. Review.
References
-
- Boonstra S, Blijleven JS, Roos WH, Onck PR, van der Giessen E, and van Oijen AM (2018) Hemagglutinin-mediated membrane fusion: A biophysical perspective, Ann. Revs. Biophys 47, 153–173. - PubMed
Publication types
MeSH terms
Substances
Grants and funding
LinkOut - more resources
Full Text Sources
Miscellaneous