Tuberous Sclerosis Complex (TSC) Inactivation Increases Neuronal Network Activity by Enhancing Ca2+ Influx via L-Type Ca2+ Channels
- PMID: 34417327
- PMCID: PMC8482857
- DOI: 10.1523/JNEUROSCI.1930-20.2021
Tuberous Sclerosis Complex (TSC) Inactivation Increases Neuronal Network Activity by Enhancing Ca2+ Influx via L-Type Ca2+ Channels
Abstract
Tuberous sclerosis complex (TSC) is a multisystem developmental disorder characterized by hamartomas in various organs, such as the brain, lungs, and kidneys. Epilepsy, along with autism and intellectual disability, is one of the neurologic impairments associated with TSC that has an intimate relationship with developmental outcomes and quality of life. Sustained activation of the mammalian target of rapamycin (mTOR) via TSC1 or TSC2 mutations is known to be involved in the onset of epilepsy in TSC. However, the mechanism by which mTOR causes seizures remains unknown. In this study, we showed that, human induced pluripotent stem cell-derived TSC2-deficient (TSC2-/-) neurons exhibited elevated neuronal activity with highly synchronized Ca2+ spikes. Notably, TSC2-/- neurons presented enhanced Ca2+ influx via L-type Ca2+ channels (LTCCs), which contributed to the abnormal neurite extension and sustained activation of cAMP response element binding protein (CREB), a critical mediator of synaptic plasticity. Expression of Cav1.3, a subtype of LTCCs, was increased in TSC2-/- neurons, but long-term rapamycin treatment suppressed this increase and reversed the altered neuronal activity and neurite extensions. Thus, we identified Cav1.3 LTCC as a critical downstream component of TSC-mTOR signaling that would trigger enhanced neuronal network activity of TSC2-/- neurons. We suggest that LTCCs could be potential novel targets for the treatment of epilepsy in TSC.SIGNIFICANCE STATEMENT There is a close relationship between elevated mammalian target of rapamycin (mTOR) activity and epilepsy in tuberous sclerosis complex (TSC). However, the underlying mechanism by which mTOR causes epilepsy remains unknown. In this study, using human TSC2-/- neurons, we identified elevated Ca2+ influx via L-type Ca2+ channels as a critical downstream component of TSC-mTOR signaling and a potential cause of both elevated neuronal activity and neurite extension in TSC2-/- neurons. Our findings demonstrate a previously unrecognized connection between sustained mTOR activation and elevated Ca2+ signaling via L-type Ca2+ channels in human TSC neurons, which could cause epilepsy in TSC.
Keywords: LTCC; TSC; calcium; epilepsy; mTOR; rapamycin.
Copyright © 2021 the authors.
Figures
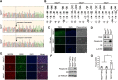
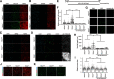
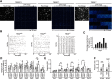
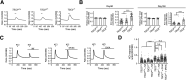
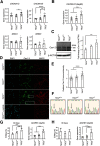
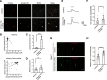
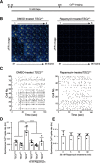
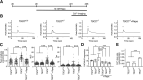
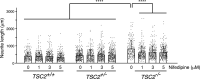
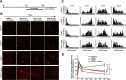
Similar articles
-
Therapeutic Approaches to Tuberous Sclerosis Complex: From Available Therapies to Promising Drug Targets.Biomolecules. 2024 Sep 21;14(9):1190. doi: 10.3390/biom14091190. Biomolecules. 2024. PMID: 39334956 Free PMC article. Review.
-
Pharmacological intervention to restore connectivity deficits of neuronal networks derived from ASD patient iPSC with a TSC2 mutation.Mol Autism. 2020 Oct 19;11(1):80. doi: 10.1186/s13229-020-00391-w. Mol Autism. 2020. PMID: 33076974 Free PMC article.
-
Biallelic Mutations in TSC2 Lead to Abnormalities Associated with Cortical Tubers in Human iPSC-Derived Neurons.J Neurosci. 2019 Nov 20;39(47):9294-9305. doi: 10.1523/JNEUROSCI.0642-19.2019. Epub 2019 Oct 7. J Neurosci. 2019. PMID: 31591157 Free PMC article.
-
The role of TSC1 and TSC2 proteins in neuronal axons.Mol Psychiatry. 2024 Apr;29(4):1165-1178. doi: 10.1038/s41380-023-02402-7. Epub 2024 Jan 11. Mol Psychiatry. 2024. PMID: 38212374 Review.
-
Tuberous sclerosis complex activity is required to control neuronal stress responses in an mTOR-dependent manner.J Neurosci. 2009 May 6;29(18):5926-37. doi: 10.1523/JNEUROSCI.0778-09.2009. J Neurosci. 2009. PMID: 19420259 Free PMC article.
Cited by
-
Mechanistic target of rapamycin signaling in human nervous system development and disease.Front Mol Neurosci. 2022 Sep 26;15:1005631. doi: 10.3389/fnmol.2022.1005631. eCollection 2022. Front Mol Neurosci. 2022. PMID: 36226315 Free PMC article. Review.
-
Potential roles of voltage-gated ion channel disruption in Tuberous Sclerosis Complex.Front Mol Neurosci. 2024 Aug 26;17:1404884. doi: 10.3389/fnmol.2024.1404884. eCollection 2024. Front Mol Neurosci. 2024. PMID: 39253727 Free PMC article.
-
Target-capture full-length double-stranded cDNA long-read sequencing through Nanopore revealed novel intron retention in patient with tuberous sclerosis complex.Front Genet. 2023 Sep 27;14:1256064. doi: 10.3389/fgene.2023.1256064. eCollection 2023. Front Genet. 2023. PMID: 37829285 Free PMC article.
-
Aberrant DJ-1 expression underlies L-type calcium channel hypoactivity in dendrites in tuberous sclerosis complex and Alzheimer's disease.Proc Natl Acad Sci U S A. 2023 Nov 7;120(45):e2301534120. doi: 10.1073/pnas.2301534120. Epub 2023 Oct 30. Proc Natl Acad Sci U S A. 2023. PMID: 37903257 Free PMC article.
-
Therapeutic Approaches to Tuberous Sclerosis Complex: From Available Therapies to Promising Drug Targets.Biomolecules. 2024 Sep 21;14(9):1190. doi: 10.3390/biom14091190. Biomolecules. 2024. PMID: 39334956 Free PMC article. Review.
References
-
- Azizan EA, Poulsen H, Tuluc P, Zhou J, Clausen MV, Lieb A, Maniero C, Garg S, Bochukova EG, Zhao W, Shaikh LH, Brighton CA, Teo AE, Davenport AP, Dekkers T, Tops B, Küsters B, Ceral J, Yeo GS, Neogi SG, et al. . (2013) Somatic mutations in ATP1A1 and CACNA1D underlie a common subtype of adrenal hypertension. Nat Genet 45:1055–1060. 10.1038/ng.2716 - DOI - PubMed
Publication types
MeSH terms
Substances
Grants and funding
LinkOut - more resources
Full Text Sources
Research Materials
Miscellaneous