Fc Galactosylation Promotes Hexamerization of Human IgG1, Leading to Enhanced Classical Complement Activation
- PMID: 34408013
- PMCID: PMC8428746
- DOI: 10.4049/jimmunol.2100399
Fc Galactosylation Promotes Hexamerization of Human IgG1, Leading to Enhanced Classical Complement Activation
Abstract
Human IgG contains one evolutionarily conserved N-linked glycan in its Fc region at position 297. This glycan is crucial for Fc-mediated functions, including its induction of the classical complement cascade. This is induced after target recognition through the IgG-Fab regions, allowing neighboring IgG-Fc tails to associate through Fc:Fc interaction, ultimately leading to hexamer formation. This hexamerization seems crucial for IgG to enable efficient interaction with the globular heads of the first complement component C1q and subsequent complement activation. In this study, we show that galactose incorporated in the IgG1-Fc enhances C1q binding, C4, C3 deposition, and complement-dependent cellular cytotoxicity in human erythrocytes and Raji cells. IgG1-Fc sialylation slightly enhanced binding of C1q, but had little effect on downstream complement activation. Using various mutations that decrease or increase hexamerization capacity of IgG1, we show that IgG1-Fc galactosylation has no intrinsic effect on C1q binding to IgG1, but enhances IgG1 hexamerization potential and, thereby, complement activation. These data suggest that the therapeutic potential of Abs can be amplified without introducing immunogenic mutations, by relatively simple glycoengineering.
Copyright © 2021 by The American Association of Immunologists, Inc.
Conflict of interest statement
The authors have no financial conflicts of interest.
Figures
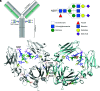
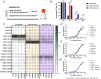
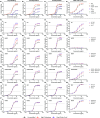
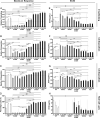
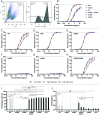
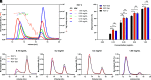
Similar articles
-
Potential of Murine IgG1 and Human IgG4 to Inhibit the Classical Complement and Fcγ Receptor Activation Pathways.Front Immunol. 2018 May 9;9:958. doi: 10.3389/fimmu.2018.00958. eCollection 2018. Front Immunol. 2018. PMID: 29867943 Free PMC article.
-
Fc galactosylation follows consecutive reaction kinetics and enhances immunoglobulin G hexamerization for complement activation.MAbs. 2021 Jan-Dec;13(1):1893427. doi: 10.1080/19420862.2021.1893427. MAbs. 2021. PMID: 33682619 Free PMC article.
-
Fc-Galactosylation of Human Immunoglobulin Gamma Isotypes Improves C1q Binding and Enhances Complement-Dependent Cytotoxicity.Front Immunol. 2017 Jun 6;8:646. doi: 10.3389/fimmu.2017.00646. eCollection 2017. Front Immunol. 2017. PMID: 28634480 Free PMC article.
-
Novel Concepts of Altered Immunoglobulin G Galactosylation in Autoimmune Diseases.Front Immunol. 2018 Mar 19;9:553. doi: 10.3389/fimmu.2018.00553. eCollection 2018. Front Immunol. 2018. PMID: 29616041 Free PMC article. Review.
-
Modular organization of proteins containing C1q-like globular domain.Immunopharmacology. 1999 May;42(1-3):15-21. doi: 10.1016/s0162-3109(99)00011-9. Immunopharmacology. 1999. PMID: 10408361 Review.
Cited by
-
Immunoglobulin G1 Fc glycosylation as an early hallmark of severe COVID-19.EBioMedicine. 2022 Apr;78:103957. doi: 10.1016/j.ebiom.2022.103957. Epub 2022 Mar 22. EBioMedicine. 2022. PMID: 35334306 Free PMC article.
-
Sialylation as an Important Regulator of Antibody Function.Front Immunol. 2022 Apr 7;13:818736. doi: 10.3389/fimmu.2022.818736. eCollection 2022. Front Immunol. 2022. PMID: 35464485 Free PMC article. Review.
-
Long-Term Culturing of FreeStyle 293-F Cells Affects Immunoglobulin G Glycome Composition.Biomolecules. 2023 Aug 14;13(8):1245. doi: 10.3390/biom13081245. Biomolecules. 2023. PMID: 37627310 Free PMC article.
-
GlYcoLISA: antigen-specific and subclass-specific IgG Fc glycosylation analysis based on an immunosorbent assay with an LC-MS readout.Nat Protoc. 2024 Jun;19(6):1887-1909. doi: 10.1038/s41596-024-00963-7. Epub 2024 Feb 21. Nat Protoc. 2024. PMID: 38383719 Review.
-
Mapping of the gene network that regulates glycan clock of ageing.Aging (Albany NY). 2023 Dec 26;15(24):14509-14552. doi: 10.18632/aging.205106. Epub 2023 Dec 26. Aging (Albany NY). 2023. PMID: 38149987 Free PMC article.
References
-
- Kapur R., Della Valle L., Verhagen O. J. H. M., Hipgrave Ederveen A., Ligthart P., de Haas M., Kumpel B., Wuhrer M., van der Schoot C. E., Vidarsson G.. 2015. Prophylactic anti-D preparations display variable decreases in Fc-fucosylation of anti-D. Transfusion 55: 553–562. - PubMed
-
- Sonneveld M. E., Natunen S., Sainio S., Koeleman C. A. M., Holst S., Dekkers G., Koelewijn J., Partanen J., van der Schoot C. E., Wuhrer M., Vidarsson G.. 2016. Glycosylation pattern of anti-platelet IgG is stable during pregnancy and predicts clinical outcome in alloimmune thrombocytopenia. Br. J. Haematol. 174: 310–320. - PubMed
Publication types
MeSH terms
Substances
LinkOut - more resources
Full Text Sources
Other Literature Sources
Miscellaneous