Determinants in Nonstructural Protein 4A of Dengue Virus Required for RNA Replication and Replication Organelle Biogenesis
- PMID: 34379504
- PMCID: PMC8513467
- DOI: 10.1128/JVI.01310-21
Determinants in Nonstructural Protein 4A of Dengue Virus Required for RNA Replication and Replication Organelle Biogenesis
Abstract
Dengue virus (DENV) constitutes one of the most important arboviral pathogens affecting humans. The high prevalence of DENV infections, which cause more than 20,000 deaths annually, and the lack of effective vaccines or direct-acting antiviral drugs make it a global health concern. DENV genome replication occurs in close association with the host endomembrane system, which is remodeled to form the viral replication organelle that originates from endoplasmic reticulum (ER) membranes. To date, the viral and cellular determinants responsible for the biogenesis of DENV replication organelles are still poorly defined. The viral nonstructural protein 4A (NS4A) can remodel membranes and has been shown to associate with numerous host factors in DENV-replicating cells. In the present study, we used reverse and forward genetic screens and identified sites within NS4A required for DENV replication. We also mapped the determinants in NS4A required for interactions with other viral proteins. Moreover, taking advantage of our recently developed polyprotein expression system, we evaluated the role of NS4A in the formation of DENV replication organelles. Together, we report a detailed map of determinants within NS4A required for RNA replication, interaction with other viral proteins, and replication organelle formation. Our results suggest that NS4A might be an attractive target for antiviral therapy. IMPORTANCE DENV is the most prevalent mosquito-borne virus, causing around 390 million infections each year. There are no approved therapies to treat DENV infection, and the only available vaccine shows limited efficacy. The viral nonstructural proteins have emerged as attractive drug targets due to their pivotal role in RNA replication and establishment of virus-induced membranous compartments, designated replication organelles (ROs). The transmembrane protein NS4A, generated by cleavage of the NS4A-2K-4B precursor, contributes to DENV replication by unknown mechanisms. Here, we report a detailed genetic interaction map of NS4A and identify residues required for RNA replication and interaction between NS4A-2K-4B and NS2B-3 as well as NS1. Importantly, by means of an expression-based system, we demonstrate the essential role of NS4A in RO biogenesis and identify determinants in NS4A required for this process. Our data suggest that NS4A is an attractive target for antiviral therapy.
Keywords: dengue virus; flavivirus; genetic mapping; membrane remodeling; nonstructural protein 4A; replication organelles.
Figures
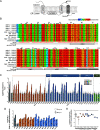
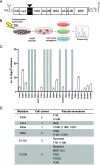
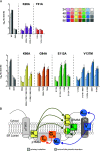
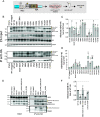
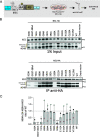
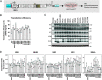
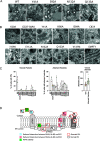
Similar articles
-
A novel interaction between dengue virus nonstructural protein 1 and the NS4A-2K-4B precursor is required for viral RNA replication but not for formation of the membranous replication organelle.PLoS Pathog. 2019 May 9;15(5):e1007736. doi: 10.1371/journal.ppat.1007736. eCollection 2019 May. PLoS Pathog. 2019. PMID: 31071189 Free PMC article.
-
A Combined Genetic-Proteomic Approach Identifies Residues within Dengue Virus NS4B Critical for Interaction with NS3 and Viral Replication.J Virol. 2015 Jul;89(14):7170-86. doi: 10.1128/JVI.00867-15. Epub 2015 Apr 29. J Virol. 2015. PMID: 25926641 Free PMC article.
-
Characterization of dengue virus NS4A and NS4B protein interaction.J Virol. 2015 Apr;89(7):3455-70. doi: 10.1128/JVI.03453-14. Epub 2015 Jan 7. J Virol. 2015. PMID: 25568208 Free PMC article.
-
Dengue Virus Non-Structural Protein 5.Viruses. 2017 Apr 24;9(4):91. doi: 10.3390/v9040091. Viruses. 2017. PMID: 28441781 Free PMC article. Review.
-
The Transactions of NS3 and NS5 in Flaviviral RNA Replication.Adv Exp Med Biol. 2018;1062:147-163. doi: 10.1007/978-981-10-8727-1_11. Adv Exp Med Biol. 2018. PMID: 29845531 Review.
Cited by
-
Computational identification of Vernonia cinerea-derived phytochemicals as potential inhibitors of nonstructural protein 1 (NSP1) in dengue virus serotype-2.Front Pharmacol. 2024 Oct 15;15:1465827. doi: 10.3389/fphar.2024.1465827. eCollection 2024. Front Pharmacol. 2024. PMID: 39474614 Free PMC article.
-
Flavivirus nonstructural proteins and replication complexes as antiviral drug targets.Curr Opin Virol. 2023 Apr;59:101305. doi: 10.1016/j.coviro.2023.101305. Epub 2023 Mar 2. Curr Opin Virol. 2023. PMID: 36870091 Free PMC article. Review.
-
Flavivirus Zika NS4A protein forms large oligomers in liposomes and in mild detergent.Sci Rep. 2024 May 31;14(1):12533. doi: 10.1038/s41598-024-63407-y. Sci Rep. 2024. PMID: 38822066 Free PMC article.
-
Nuclear membrane protein SUN2 promotes replication of flaviviruses through modulating cytoskeleton reorganization mediated by NS1.Nat Commun. 2024 Jan 5;15(1):296. doi: 10.1038/s41467-023-44580-6. Nat Commun. 2024. PMID: 38177122 Free PMC article.
-
Identification of RACK1 as a novel regulator of non-structural protein 4 of chikungunya virus.Acta Biochim Biophys Sin (Shanghai). 2024 May 29;56(10):1425-1436. doi: 10.3724/abbs.2024073. Acta Biochim Biophys Sin (Shanghai). 2024. PMID: 38813597 Free PMC article.
References
-
- Bhatt S, Gething PW, Brady OJ, Messina JP, Farlow AW, Moyes CL, Drake JM, Brownstein JS, Hoen AG, Sankoh O, Myers MF, George DB, Jaenisch T, Wint GR, Simmons CP, Scott TW, Farrar JJ, Hay SI. 2013. The global distribution and burden of dengue. Nature 496:504–507. 10.1038/nature12060. - DOI - PMC - PubMed
-
- Hadinegoro SR, Arredondo-Garcia JL, Capeding MR, Deseda C, Chotpitayasunondh T, Dietze R, Muhammad Ismail HI, Reynales H, Limkittikul K, Rivera-Medina DM, Tran HN, Bouckenooghe A, Chansinghakul D, Cortes M, Fanouillere K, Forrat R, Frago C, Gailhardou S, Jackson N, Noriega F, Plennevaux E, Wartel TA, Zambrano B, Saville M, CYD-TDV Dengue Vaccine Working Group. 2015. Efficacy and long-term safety of a dengue vaccine in regions of endemic disease. N Engl J Med 373:1195–1206. 10.1056/NEJMoa1506223. - DOI - PubMed
-
- Płaszczyca A, Scaturro P, Neufeldt CJ, Cortese M, Cerikan B, Ferla S, Brancale A, Pichlmair A, Bartenschlager R. 2019. A novel interaction between dengue virus nonstructural protein 1 and the NS4A-2K-4B precursor is required for viral RNA replication but not for formation of the membranous replication organelle. PLoS Pathog 15:e1007736. 10.1371/journal.ppat.1007736. - DOI - PMC - PubMed
Publication types
MeSH terms
Substances
Grants and funding
LinkOut - more resources
Full Text Sources
Medical
Research Materials