Future Match Making: When Pediatric Oncology Meets Organoid Technology
- PMID: 34327198
- PMCID: PMC8315550
- DOI: 10.3389/fcell.2021.674219
Future Match Making: When Pediatric Oncology Meets Organoid Technology
Abstract
Unlike adult cancers that frequently result from the accumulation in time of mutational "hits" often linked to lifestyle, childhood cancers are emerging as diseases of dysregulated development through massive epigenetic alterations. The ability to reconstruct these differences in cancer models is therefore crucial for better understanding the uniqueness of pediatric cancer biology. Cancer organoids (i.e., tumoroids) represent a promising approach for creating patient-derived in vitro cancer models that closely recapitulate the overall pathophysiological features of natural tumorigenesis, including intra-tumoral heterogeneity and plasticity. Though largely applied to adult cancers, this technology is scarcely used for childhood cancers, with a notable delay in technological transfer. However, tumoroids could provide an unprecedented tool to unravel the biology of pediatric cancers and improve their therapeutic management. We herein present the current state-of-the-art of a long awaited and much needed matchmaking.
Keywords: cancer; genetic engineering; heterogeneity; modeling; organoids; pediatric cancer and oncology; plasticity; tumoroids.
Copyright © 2021 Barbet and Broutier.
Conflict of interest statement
The authors declare that the research was conducted in the absence of any commercial or financial relationships that could be construed as a potential conflict of interest.
Figures
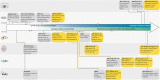
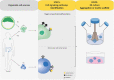
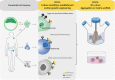
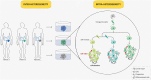

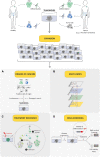

Similar articles
-
Cancer modeling meets human organoid technology.Science. 2019 Jun 7;364(6444):952-955. doi: 10.1126/science.aaw6985. Science. 2019. PMID: 31171691
-
Organoids: An intermediate modeling platform in precision oncology.Cancer Lett. 2018 Feb 1;414:174-180. doi: 10.1016/j.canlet.2017.11.021. Epub 2017 Nov 22. Cancer Lett. 2018. PMID: 29174804 Review.
-
A synopsis of prostate organoid methodologies, applications, and limitations.Prostate. 2020 May;80(6):518-526. doi: 10.1002/pros.23966. Epub 2020 Feb 21. Prostate. 2020. PMID: 32084293 Review.
-
How well do brain organoids capture your brain?iScience. 2021 Jan 19;24(2):102063. doi: 10.1016/j.isci.2021.102063. eCollection 2021 Feb 19. iScience. 2021. PMID: 33554067 Free PMC article. Review.
-
Intestinal organoids: A new paradigm for engineering intestinal epithelium in vitro.Biomaterials. 2019 Feb;194:195-214. doi: 10.1016/j.biomaterials.2018.12.006. Epub 2018 Dec 10. Biomaterials. 2019. PMID: 30612006 Review.
Cited by
-
Pediatric Tumors as Disorders of Development: The Case for In Vitro Modeling Based on Human Stem Cells.Cancer Control. 2024 Jan-Dec;31:10732748241270564. doi: 10.1177/10732748241270564. Cancer Control. 2024. PMID: 39118322 Free PMC article. Review.
-
Organoids as a new approach for improving pediatric cancer research.Front Oncol. 2024 May 21;14:1414311. doi: 10.3389/fonc.2024.1414311. eCollection 2024. Front Oncol. 2024. PMID: 38835365 Free PMC article. Review.
-
In Vitro Models of Head and Neck Cancer: From Primitive to Most Advanced.J Pers Med. 2023 Nov 3;13(11):1575. doi: 10.3390/jpm13111575. J Pers Med. 2023. PMID: 38003890 Free PMC article. Review.
-
Patient-derived tumor organoids: a new avenue for preclinical research and precision medicine in oncology.Exp Mol Med. 2024 Jul;56(7):1531-1551. doi: 10.1038/s12276-024-01272-5. Epub 2024 Jul 1. Exp Mol Med. 2024. PMID: 38945959 Free PMC article. Review.
-
Pan-cancer landscape of aberrant DNA Methylation across childhood Cancers: Molecular Characteristics and Clinical relevance.Exp Hematol Oncol. 2022 Nov 8;11(1):89. doi: 10.1186/s40164-022-00339-1. Exp Hematol Oncol. 2022. PMID: 36348462 Free PMC article.
References
Publication types
LinkOut - more resources
Full Text Sources