Integrated stress response control of granulosa cell translation and proliferation during normal ovarian follicle development
- PMID: 34314477
- PMCID: PMC8660582
- DOI: 10.1093/molehr/gaab050
Integrated stress response control of granulosa cell translation and proliferation during normal ovarian follicle development
Abstract
Mechanisms that directly control mammalian ovarian primordial follicle (PF) growth activation and the selection of individual follicles for survival are largely unknown. Follicle cells produce factors that can act as potent inducers of cellular stress during normal function. Consistent with this, we show here that normal, untreated ovarian cells, including pre-granulosa cells of dormant PFs, express phenotype and protein markers of the activated integrated stress response (ISR), including stress-specific protein translation (phospho-Serine 51 eukaryotic initiation factor 2α; P-EIF2α), active DNA damage checkpoints, and cell-cycle arrest. We further demonstrate that mRNAs upregulated in primary (growing) follicles versus arrested PFs mostly include stress-responsive upstream open reading frames (uORFs). Treatment of a granulosa cell (GC) line with the PF growth trigger tumor necrosis factor alpha results in the upregulation of a 'stress-dependent' translation profile. This includes further elevated P-eIF2α and a shift of uORF-containing mRNAs to polysomes. Because the active ISR corresponds to slow follicle growth and PF arrest, we propose that repair and abrogation of ISR checkpoints (e.g. checkpoint recovery) drives the GC cell cycle and PF growth activation (PFGA). If cellular stress is elevated beyond a threshold(s) or, if damage occurs that cannot be repaired, cell and follicle death ensue, consistent with physiological atresia. These data suggest an intrinsic quality control mechanism for immature and growing follicles, where PFGA and subsequent follicle growth and survival depend causally upon ISR resolution, including DNA repair and thus the proof of genomic integrity.
Keywords: aging; eukaryotic initiation Factor 2 (EIF2); follicle; integrated stress response; menopause; oocyte; ovary; translational control.
© The Author(s) 2021. Published by Oxford University Press on behalf of European Society of Human Reproduction and Embryology. All rights reserved. For permissions, please email: journals.permissions@oup.com.
Figures
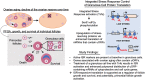
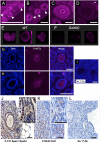
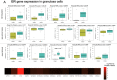
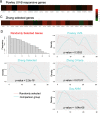
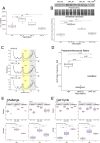
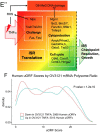
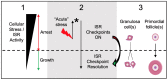
Comment in
-
Primordial follicle activation: to be or not to be takes another twist.Biol Reprod. 2022 Apr 26;106(4):639-641. doi: 10.1093/biolre/ioac033. Biol Reprod. 2022. PMID: 35243492 No abstract available.
Similar articles
-
Recapitulating human ovarian aging using random walks.PeerJ. 2022 Aug 22;10:e13941. doi: 10.7717/peerj.13941. eCollection 2022. PeerJ. 2022. PMID: 36032944 Free PMC article.
-
Granulosa cells from human primordial and primary follicles show differential global gene expression profiles.Hum Reprod. 2018 Apr 1;33(4):666-679. doi: 10.1093/humrep/dey011. Hum Reprod. 2018. PMID: 29506120
-
An upstream open reading frame regulates translation of GADD34 during cellular stresses that induce eIF2alpha phosphorylation.J Biol Chem. 2009 Mar 13;284(11):6661-73. doi: 10.1074/jbc.M806735200. Epub 2009 Jan 8. J Biol Chem. 2009. PMID: 19131336 Free PMC article.
-
Beyond apoptosis: evidence of other regulated cell death pathways in the ovary throughout development and life.Hum Reprod Update. 2023 Jul 5;29(4):434-456. doi: 10.1093/humupd/dmad005. Hum Reprod Update. 2023. PMID: 36857094 Free PMC article. Review.
-
Upstream Open Reading Frames Differentially Regulate Gene-specific Translation in the Integrated Stress Response.J Biol Chem. 2016 Aug 12;291(33):16927-35. doi: 10.1074/jbc.R116.733899. Epub 2016 Jun 29. J Biol Chem. 2016. PMID: 27358398 Free PMC article. Review.
Cited by
-
Harnessing the Power of Purple Sweet Potato Color and Myo-Inositol to Treat Classic Galactosemia.Int J Mol Sci. 2022 Aug 4;23(15):8654. doi: 10.3390/ijms23158654. Int J Mol Sci. 2022. PMID: 35955788 Free PMC article.
-
Recapitulating human ovarian aging using random walks.PeerJ. 2022 Aug 22;10:e13941. doi: 10.7717/peerj.13941. eCollection 2022. PeerJ. 2022. PMID: 36032944 Free PMC article.
-
Spatio-temporal remodelling of the composition and architecture of the human ovarian cortical extracellular matrix during in vitro culture.Hum Reprod. 2023 Mar 1;38(3):444-458. doi: 10.1093/humrep/dead008. Hum Reprod. 2023. PMID: 36721914 Free PMC article.
-
The Aging Ovary and the Tales Learned Since Fetal Development.Sex Dev. 2023;17(2-3):156-168. doi: 10.1159/000532072. Epub 2023 Aug 18. Sex Dev. 2023. PMID: 37598664 Free PMC article. Review.
-
Early postnatal alterations in follicular stress response and survival in a mouse model of Classic Galactosemia.J Ovarian Res. 2022 Nov 21;15(1):122. doi: 10.1186/s13048-022-01049-2. J Ovarian Res. 2022. PMID: 36414970 Free PMC article.
References
-
- Balakrishnan B, Nicholas C, Siddiqi A, Chen W, Bales E, Feng M, Johnson J, Lai K. Reversal of aberrant PI3K/Akt signaling by Salubrinal in a GalT-deficient mouse model. Biochim Biophys Acta Mol Basis Dis 2017;1863:3286–3293. - PubMed
Publication types
MeSH terms
Substances
Associated data
Grants and funding
LinkOut - more resources
Full Text Sources
Miscellaneous