Advances in 3D-Printed Surface-Modified Ca-Si Bioceramic Structures and Their Potential for Bone Tumor Therapy
- PMID: 34300763
- PMCID: PMC8306413
- DOI: 10.3390/ma14143844
Advances in 3D-Printed Surface-Modified Ca-Si Bioceramic Structures and Their Potential for Bone Tumor Therapy
Abstract
Bioceramics such as calcium silicate (Ca-Si), have gained a lot of interest in the biomedical field due to their strength, osteogenesis capability, mechanical stability, and biocompatibility. As such, these materials are excellent candidates to promote bone and tissue regeneration along with treating bone cancer. Bioceramic scaffolds, functionalized with appropriate materials, can achieve desirable photothermal effects, opening up a bifunctional approach to osteosarcoma treatments-simultaneously killing cancerous cells while expediting healthy bone tissue regeneration. At the same time, they can also be used as vehicles and cargo structures to deliver anticancer drugs and molecules in a targeted manner to tumorous tissue. However, the traditional synthesis routes for these bioceramic scaffolds limit the macro-, micro-, and nanostructures necessary for maximal benefits for photothermal therapy and drug delivery. Therefore, a different approach to formulate bioceramic scaffolds has emerged in the form of 3D printing, which offers a sustainable, highly reproducible, and scalable method for the production of valuable biomedical materials. Here, calcium silicate (Ca-Si) is reviewed as a novel 3D printing base material, functionalized with highly photothermal materials for osteosarcoma therapy and drug delivery platforms. Consequently, this review aims to detail advances made towards functionalizing 3D-printed Ca-Si and similar bioceramic scaffold structures as well as their resulting applications for various aspects of tumor therapy, with a focus on the external surface and internal dispersion functionalization of the scaffolds.
Keywords: bioceramics; biocompatibility; calcium silicate; cancer therapy; nanomaterials; osteosarcoma; photothermal therapy.
Conflict of interest statement
The authors declare no conflict of interest.
Figures
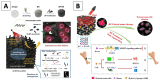
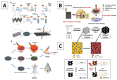
Similar articles
-
3D-printed bioceramic scaffolds: From bone tissue engineering to tumor therapy.Acta Biomater. 2018 Oct 1;79:37-59. doi: 10.1016/j.actbio.2018.08.026. Epub 2018 Aug 28. Acta Biomater. 2018. PMID: 30165201 Review.
-
Appreciable biosafety, biocompatibility and osteogenic capability of 3D printed nonstoichiometric wollastonite scaffolds favorable for clinical translation.J Orthop Translat. 2024 Mar 13;45:88-99. doi: 10.1016/j.jot.2024.02.004. eCollection 2024 Mar. J Orthop Translat. 2024. PMID: 38516038 Free PMC article.
-
Fabrication and in vitro evaluation of 3D printed porous silicate substituted calcium phosphate scaffolds for bone tissue engineering.Biotechnol Bioeng. 2022 Nov;119(11):3297-3310. doi: 10.1002/bit.28202. Epub 2022 Aug 13. Biotechnol Bioeng. 2022. PMID: 35923072
-
Multifunctional 3D-printed bioceramic scaffolds: Recent strategies for osteosarcoma treatment.J Tissue Eng. 2023 May 15;14:20417314231170371. doi: 10.1177/20417314231170371. eCollection 2023 Jan-Dec. J Tissue Eng. 2023. PMID: 37205149 Free PMC article. Review.
-
3D printing of biomaterials with mussel-inspired nanostructures for tumor therapy and tissue regeneration.Biomaterials. 2016 Dec;111:138-148. doi: 10.1016/j.biomaterials.2016.10.005. Epub 2016 Oct 5. Biomaterials. 2016. PMID: 27728813
Cited by
-
Functional engineering strategies of 3D printed implants for hard tissue replacement.Regen Biomater. 2022 Nov 24;10:rbac094. doi: 10.1093/rb/rbac094. eCollection 2023. Regen Biomater. 2022. PMID: 36683758 Free PMC article. Review.
-
Trends of calcium silicate biomaterials in medical research and applications: A bibliometric analysis from 1990 to 2020.Front Pharmacol. 2022 Oct 14;13:991377. doi: 10.3389/fphar.2022.991377. eCollection 2022. Front Pharmacol. 2022. PMID: 36313285 Free PMC article.
-
Multifunctional inorganic biomaterials: New weapons targeting osteosarcoma.Front Mol Biosci. 2023 Jan 4;9:1105540. doi: 10.3389/fmolb.2022.1105540. eCollection 2022. Front Mol Biosci. 2023. PMID: 36660426 Free PMC article. Review.
References
-
- Evans D.R., Lazarides A.L., Visgauss J.D., Somarelli J.A., Blazer D.G., Brigman B.E., Eward W.C. Limb salvage versus amputation in patients with osteosarcoma of the extremities: An update in the modern era using the National Cancer Database. BMC Cancer. 2020;20:995. doi: 10.1186/s12885-020-07502-z. - DOI - PMC - PubMed
Publication types
Grants and funding
LinkOut - more resources
Full Text Sources
Research Materials